Search This Blog
Buyer behaviour.
Deciphering Buying Behaviour & Consumer Lifestyles for Marketing Decisions.

Prior Hypothesis Bias
Popular posts, common man's cars, world peace, yes; info. to the world, no.
Confirmation Bias In Psychology: Definition & Examples
Julia Simkus
Editor at Simply Psychology
BA (Hons) Psychology, Princeton University
Julia Simkus is a graduate of Princeton University with a Bachelor of Arts in Psychology. She is currently studying for a Master's Degree in Counseling for Mental Health and Wellness in September 2023. Julia's research has been published in peer reviewed journals.
Learn about our Editorial Process
Saul McLeod, PhD
Editor-in-Chief for Simply Psychology
BSc (Hons) Psychology, MRes, PhD, University of Manchester
Saul McLeod, PhD., is a qualified psychology teacher with over 18 years of experience in further and higher education. He has been published in peer-reviewed journals, including the Journal of Clinical Psychology.
On This Page:
Confirmation Bias is the tendency to look for information that supports, rather than rejects, one’s preconceptions, typically by interpreting evidence to confirm existing beliefs while rejecting or ignoring any conflicting data (American Psychological Association).
One of the early demonstrations of confirmation bias appeared in an experiment by Peter Watson (1960) in which the subjects were to find the experimenter’s rule for sequencing numbers.
Its results showed that the subjects chose responses that supported their hypotheses while rejecting contradictory evidence, and even though their hypotheses were incorrect, they became confident in them quickly (Gray, 2010, p. 356).
Though such evidence of confirmation bias has appeared in psychological literature throughout history, the term ‘confirmation bias’ was first used in a 1977 paper detailing an experimental study on the topic (Mynatt, Doherty, & Tweney, 1977).
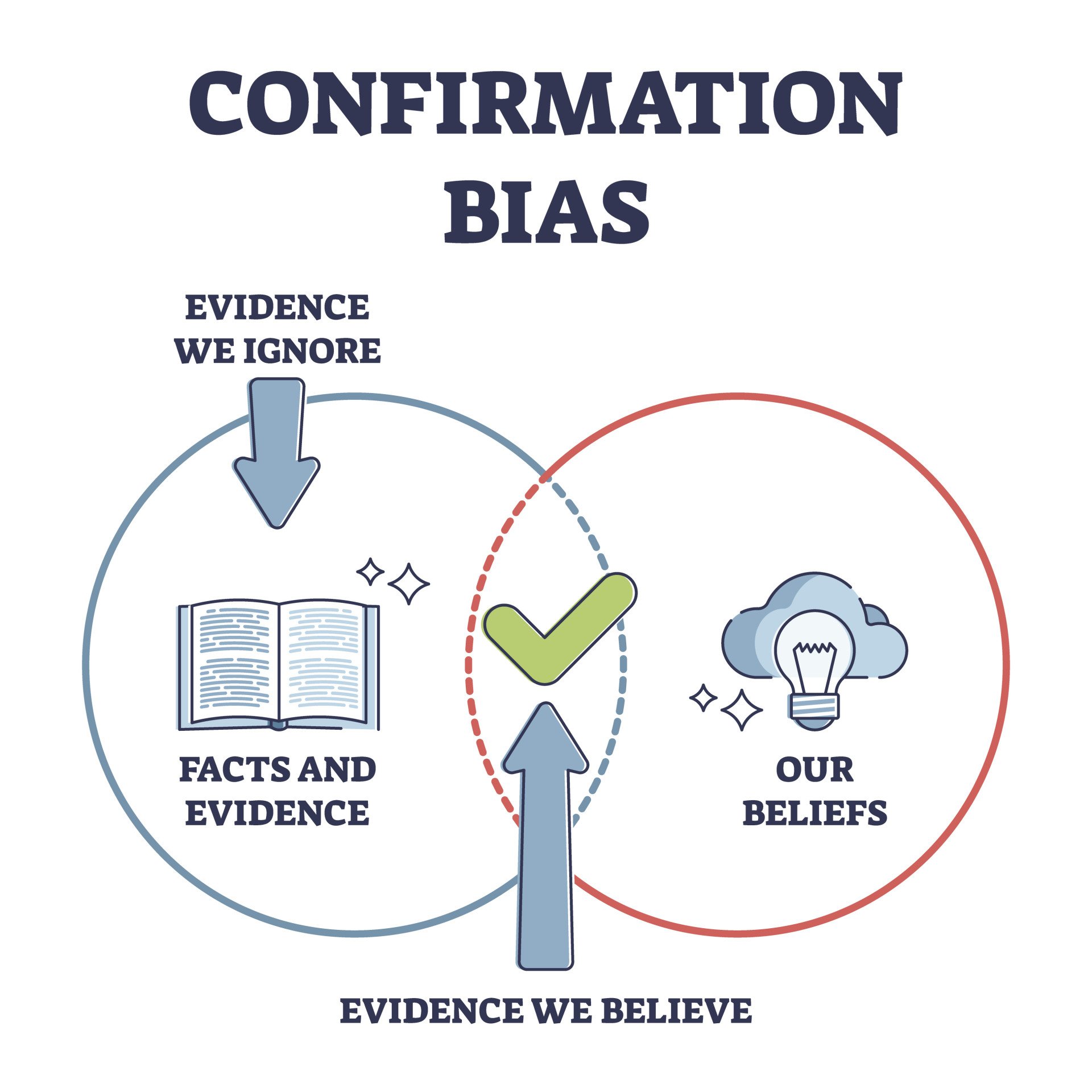
Biased Search for Information
This type of confirmation bias explains people’s search for evidence in a one-sided way to support their hypotheses or theories.
Experiments have shown that people provide tests/questions designed to yield “yes” if their favored hypothesis is true and ignore alternative hypotheses that are likely to give the same result.
This is also known as the congruence heuristic (Baron, 2000, p.162-64). Though the preference for affirmative questions itself may not be biased, there are experiments that have shown that congruence bias does exist.
For Example:
If you were to search “Are cats better than dogs?” in Google, all you would get are sites listing the reasons why cats are better.
However, if you were to search “Are dogs better than cats?” google will only provide you with sites that believe dogs are better than cats.
This shows that phrasing questions in a one-sided way (i.e., affirmative manner) will assist you in obtaining evidence consistent with your hypothesis.
Biased Interpretation
This type of bias explains that people interpret evidence concerning their existing beliefs by evaluating confirming evidence differently than evidence that challenges their preconceptions.
Various experiments have shown that people tend not to change their beliefs on complex issues even after being provided with research because of the way they interpret the evidence.
Additionally, people accept “confirming” evidence more easily and critically evaluate the “disconfirming” evidence (this is known as disconfirmation bias) (Taber & Lodge, 2006).
When provided with the same evidence, people’s interpretations could still be biased.
For example:
Biased interpretation is shown in an experiment conducted by Stanford University on the topic of capital punishment. It included participants who were in support of and others who were against capital punishment.
All subjects were provided with the same two studies.
After reading the detailed descriptions of the studies, participants still held their initial beliefs and supported their reasoning by providing “confirming” evidence from the studies and rejecting any contradictory evidence or considering it inferior to the “confirming” evidence (Lord, Ross, & Lepper, 1979).
Biased Memory
To confirm their current beliefs, people may remember/recall information selectively. Psychological theories vary in defining memory bias.
Some theories state that information confirming prior beliefs is stored in the memory while contradictory evidence is not (i.e., Schema theory). Some others claim that striking information is remembered best (i.e., humor effect).
Memory confirmation bias also serves a role in stereotype maintenance. Experiments have shown that the mental association between expectancy-confirming information and the group label strongly affects recall and recognition memory.
Though a certain stereotype about a social group might not be true for an individual, people tend to remember the stereotype-consistent information better than any disconfirming evidence (Fyock & Stangor, 1994).
In one experimental study, participants were asked to read a woman’s profile (detailing her extroverted and introverted skills) and assess her for either a job of a librarian or real-estate salesperson.
Those assessing her as a salesperson better recalled extroverted traits, while the other group recalled more examples of introversion (Snyder & Cantor, 1979).
These experiments, along with others, have offered an insight into selective memory and provided evidence for biased memory, proving that one searches for and better remembers confirming evidence.
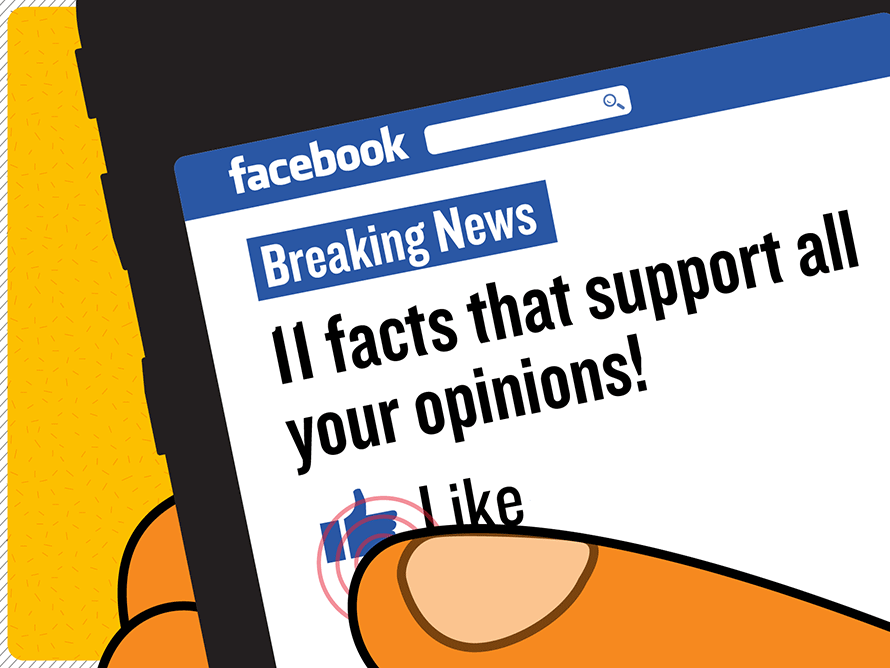
Social Media
Information we are presented on social media is not only reflective of what the users want to see but also of the designers’ beliefs and values. Today, people are exposed to an overwhelming number of news sources, each varying in their credibility.
To form conclusions, people tend to read the news that aligns with their perspectives. For instance, new channels provide information (even the same news) differently from each other on complex issues (i.e., racism, political parties, etc.), with some using sensational headlines/pictures and one-sided information.
Due to the biased coverage of topics, people only utilize certain channels/sites to obtain their information to make biased conclusions.
Religious Faith
People also tend to search for and interpret evidence with respect to their religious beliefs (if any).
For instance, on the topics of abortion and transgender rights, people whose religions are against such things will interpret this information differently than others and will look for evidence to validate what they believe.
Similarly, those who religiously reject the theory of evolution will either gather information disproving evolution or hold no official stance on the topic.
Also, irreligious people might perceive events that are considered “miracles” and “test of faiths” by religious people to be a reinforcement of their lack of faith in a religion.
when Does The Confirmation Bias Occur?
There are several explanations why humans possess confirmation bias, including this tendency being an efficient way to process information, protect self-esteem, and minimize cognitive dissonance.
Information Processing
Confirmation bias serves as an efficient way to process information because of the limitless information humans are exposed to.
To form an unbiased decision, one would have to critically evaluate every piece of information present, which is unfeasible. Therefore, people only tend to look for information desired to form their conclusions (Casad, 2019).
Protect Self-esteem
People are susceptible to confirmation bias to protect their self-esteem (to know that their beliefs are accurate).
To make themselves feel confident, they tend to look for information that supports their existing beliefs (Casad, 2019).
Minimize Cognitive Dissonance
Cognitive dissonance also explains why confirmation bias is adaptive.
Cognitive dissonance is a mental conflict that occurs when a person holds two contradictory beliefs and causes psychological stress/unease in a person.
To minimize this dissonance, people adapt to confirmation bias by avoiding information that is contradictory to their views and seeking evidence confirming their beliefs.
Challenge avoidance and reinforcement seeking to affect people’s thoughts/reactions differently since exposure to disconfirming information results in negative emotions, something that is nonexistent when seeking reinforcing evidence (“The Confirmation Bias: Why People See What They Want to See”).
Implications
Confirmation bias consistently shapes the way we look for and interpret information that influences our decisions in this society, ranging from homes to global platforms. This bias prevents people from gathering information objectively.
During the election campaign, people tend to look for information confirming their perspectives on different candidates while ignoring any information contradictory to their views.
This subjective manner of obtaining information can lead to overconfidence in a candidate, and misinterpretation/overlooking of important information, thus influencing their voting decision and, eventually country’s leadership (Cherry, 2020).
Recruitment and Selection
Confirmation bias also affects employment diversity because preconceived ideas about different social groups can introduce discrimination (though it might be unconscious) and impact the recruitment process (Agarwal, 2018).
Existing beliefs of a certain group being more competent than the other is the reason why particular races and gender are represented the most in companies today. This bias can hamper the company’s attempt at diversifying its employees.
Mitigating Confirmation Bias
Change in intrapersonal thought:.
To avoid being susceptible to confirmation bias, start questioning your research methods, and sources used to obtain their information.
Expanding the types of sources used in searching for information could provide different aspects of a particular topic and offer levels of credibility.
- Read entire articles rather than forming conclusions based on the headlines and pictures. – Search for credible evidence presented in the article.
- Analyze if the statements being asserted are backed up by trustworthy evidence (tracking the source of evidence could prove its credibility). – Encourage yourself and others to gather information in a conscious manner.
Alternative hypothesis:
Confirmation bias occurs when people tend to look for information that confirms their beliefs/hypotheses, but this bias can be reduced by taking into alternative hypotheses and their consequences.
Considering the possibility of beliefs/hypotheses other than one’s own could help you gather information in a more dynamic manner (rather than a one-sided way).
Related Cognitive Biases
There are many cognitive biases that characterize as subtypes of confirmation bias. Following are two of the subtypes:
Backfire Effect
The backfire effect occurs when people’s preexisting beliefs strengthen when challenged by contradictory evidence (Silverman, 2011).
- Therefore, disproving a misconception can actually strengthen a person’s belief in that misconception.
One piece of disconfirming evidence does not change people’s views, but a constant flow of credible refutations could correct misinformation/misconceptions.
This effect is considered a subtype of confirmation bias because it explains people’s reactions to new information based on their preexisting hypotheses.
A study by Brendan Nyhan and Jason Reifler (two researchers on political misinformation) explored the effects of different types of statements on people’s beliefs.
While examining two statements, “I am not a Muslim, Obama says.” and “I am a Christian, Obama says,” they concluded that the latter statement is more persuasive and resulted in people’s change of beliefs, thus affirming statements are more effective at correcting incorrect views (Silverman, 2011).
Halo Effect
The halo effect occurs when people use impressions from a single trait to form conclusions about other unrelated attributes. It is heavily influenced by the first impression.
Research on this effect was pioneered by American psychologist Edward Thorndike who, in 1920, described ways officers rated their soldiers on different traits based on first impressions (Neugaard, 2019).
Experiments have shown that when positive attributes are presented first, a person is judged more favorably than when negative traits are shown first. This is a subtype of confirmation bias because it allows us to structure our thinking about other information using only initial evidence.
Learning Check
When does the confirmation bias occur.
- When an individual only researches information that is consistent with personal beliefs.
- When an individual only makes a decision after all perspectives have been evaluated.
- When an individual becomes more confident in one’s judgments after researching alternative perspectives.
- When an individual believes that the odds of an event occurring increase if the event hasn’t occurred recently.
The correct answer is A. Confirmation bias occurs when an individual only researches information consistent with personal beliefs. This bias leads people to favor information that confirms their preconceptions or hypotheses, regardless of whether the information is true.
Take-home Messages
- Confirmation bias is the tendency of people to favor information that confirms their existing beliefs or hypotheses.
- Confirmation bias happens when a person gives more weight to evidence that confirms their beliefs and undervalues evidence that could disprove it.
- People display this bias when they gather or recall information selectively or when they interpret it in a biased way.
- The effect is stronger for emotionally charged issues and for deeply entrenched beliefs.
Agarwal, P., Dr. (2018, October 19). Here Is How Bias Can Affect Recruitment In Your Organisation. https://www.forbes.com/sites/pragyaagarwaleurope/2018/10/19/how-can-bias-during-interviewsaffect-recruitment-in-your-organisation
American Psychological Association. (n.d.). APA Dictionary of Psychology. https://dictionary.apa.org/confirmation-bias
Baron, J. (2000). Thinking and Deciding (Third ed.). Cambridge University Press.
Casad, B. (2019, October 09). Confirmation bias . https://www.britannica.com/science/confirmation-bias
Cherry, K. (2020, February 19). Why Do We Favor Information That Confirms Our Existing Beliefs? https://www.verywellmind.com/what-is-a-confirmation-bias-2795024
Fyock, J., & Stangor, C. (1994). The role of memory biases in stereotype maintenance. The British journal of social psychology, 33 (3), 331–343.
Gray, P. O. (2010). Psychology . New York: Worth Publishers.
Lord, C. G., Ross, L., & Lepper, M. R. (1979). Biased assimilation and attitude polarization: The effects of prior theories on subsequently considered evidence. Journal of Personality and Social Psychology, 37 (11), 2098–2109.
Mynatt, C. R., Doherty, M. E., & Tweney, R. D. (1977). Confirmation bias in a simulated research environment: An experimental study of scientific inference. Quarterly Journal of Experimental Psychology, 29 (1), 85-95.
Neugaard, B. (2019, October 09). Halo effect. https://www.britannica.com/science/halo-effect
Silverman, C. (2011, June 17). The Backfire Effect . https://archives.cjr.org/behind_the_news/the_backfire_effect.php
Snyder, M., & Cantor, N. (1979). Testing hypotheses about other people: The use of historical knowledge. Journal of Experimental Social Psychology, 15 (4), 330–342.
Further Information
- What Is Confirmation Bias and When Do People Actually Have It?
- Confirmation Bias: A Ubiquitous Phenomenon in Many Guises
- The importance of making assumptions: why confirmation is not necessarily a bias
- Decision Making Is Caused By Information Processing And Emotion: A Synthesis Of Two Approaches To Explain The Phenomenon Of Confirmation Bias
Confirmation bias occurs when individuals selectively collect, interpret, or remember information that confirms their existing beliefs or ideas, while ignoring or discounting evidence that contradicts these beliefs.
This bias can happen unconsciously and can influence decision-making and reasoning in various contexts, such as research, politics, or everyday decision-making.
What is confirmation bias in psychology?
Confirmation bias in psychology is the tendency to favor information that confirms existing beliefs or values. People exhibiting this bias are likely to seek out, interpret, remember, and give more weight to evidence that supports their views, while ignoring, dismissing, or undervaluing the relevance of evidence that contradicts them.
This can lead to faulty decision-making because one-sided information doesn’t provide a full picture.
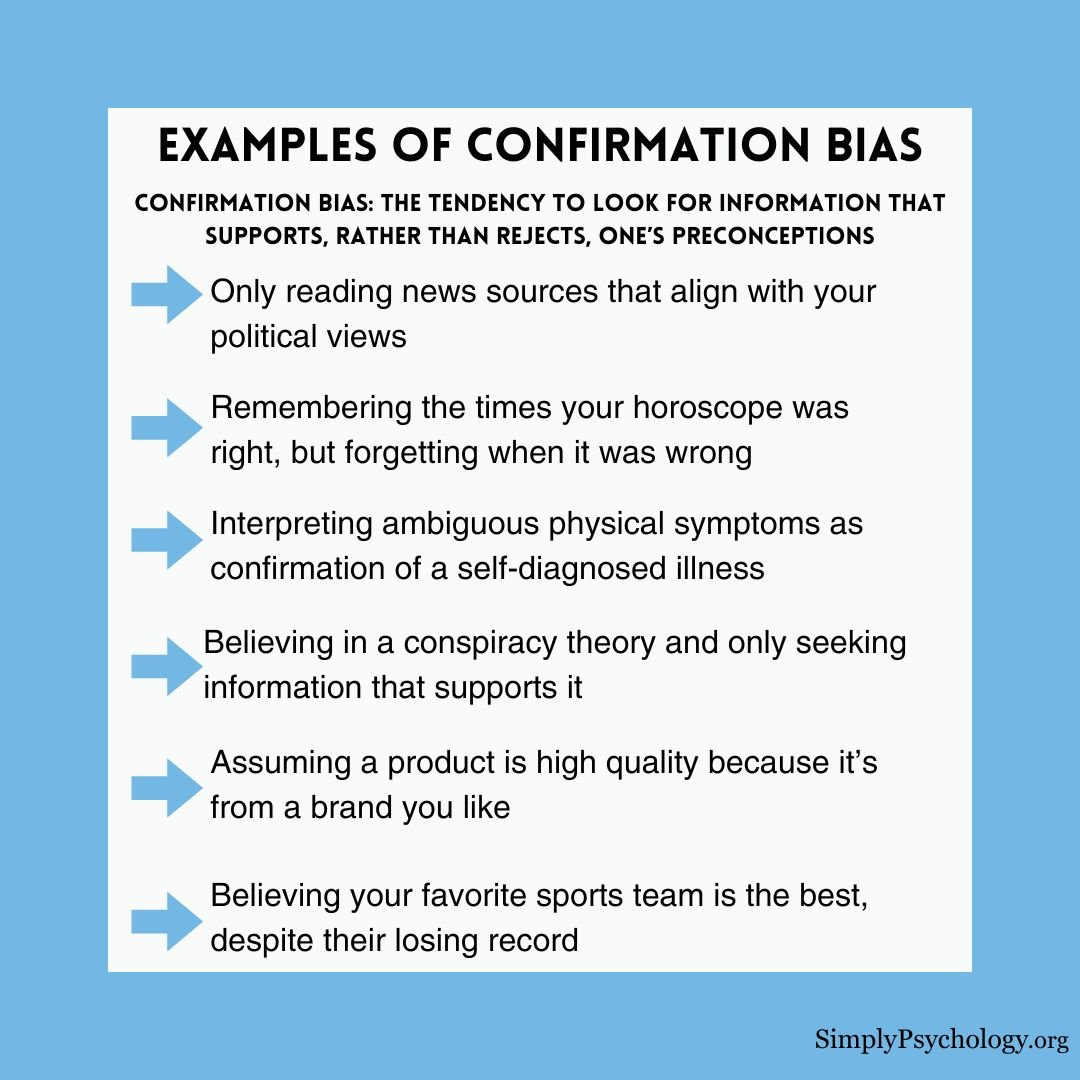
An official website of the United States government
Official websites use .gov A .gov website belongs to an official government organization in the United States.
Secure .gov websites use HTTPS A lock ( Lock Locked padlock icon ) or https:// means you've safely connected to the .gov website. Share sensitive information only on official, secure websites.
- Publications
- Account settings
- Advanced Search
- Journal List

How prior preferences determine decision-making frames and biases in the human brain
Alizée lopez-persem, philippe domenech, mathias pessiglione.
- Author information
- Article notes
- Copyright and License information
Email: [email protected]
Received 2016 Aug 6; Accepted 2016 Nov 14; Collection date 2016.
This article is distributed under the terms of the Creative Commons Attribution License , which permits unrestricted use and redistribution provided that the original author and source are credited.
Understanding how option values are compared when making a choice is a key objective for decision neuroscience. In natural situations, agents may have a priori on their preferences that create default policies and shape the neural comparison process. We asked participants to make choices between items belonging to different categories (e.g., jazz vs. rock music). Behavioral data confirmed that the items taken from the preferred category were chosen more often and more rapidly, which qualified them as default options. FMRI data showed that baseline activity in classical brain valuation regions, such as the ventromedial Prefrontal Cortex (vmPFC), reflected the strength of prior preferences. In addition, evoked activity in the same regions scaled with the default option value, irrespective of the eventual choice. We therefore suggest that in the brain valuation system, choices are framed as comparisons between default and alternative options, which might save some resource but induce a decision bias.
DOI: http://dx.doi.org/10.7554/eLife.20317.001
Research Organism: Human
eLife digest
If you had the choice of listening to a piece of music by either the singer Céline Dion or jazz pianist Keith Jarrett, which would you pick? When choosing between two mutually exclusive options, the brain first assigns a value to each. An area called the ventromedial prefrontal cortex (vmPFC) compares these two values and calculates the difference between them. The vmPFC then relays this difference to other brain regions that trigger the movements required to obtain the selected option.
But what exactly is the vmPFC comparing? A reasonable assumption is that we approach the decision with an existing preference for one of the options based on our previous experience. Lopez-Persem et al. set out to determine whether and how the vmPFC uses this existing preference – for example, for pop music over jazz – to drive the decision-making process.
For the experiments, volunteers were asked to rate how much they liked individual musicians spanning a range of different genres. While lying inside a brain scanner, the subjects then had to choose their favorite from pairs of musicians selected from the list. When making such decisions, volunteers must consider both the overall category (do I prefer jazz or pop?) but also the individual examples (a pop music fan might choose jazz if the pop option is Britney Spears). Lopez-Persem et al. found that the volunteer’s decisions were biased towards their prior preference. Pop music fans chose Céline Dion or Britney Spears more often than would be expected based on the likability ratings they had given the individual artists in the study.
Brain imaging revealed that the vmPFC represents choices as ‘default minus alternative’, where the default is any member of the previously preferred category (e.g. any pop artist for a pop music fan) and the alternative is from a different category (e.g. a jazz artist). Baseline vmPFC activity is higher for members of the preferred category, giving these options a head start over the alternatives. Asking volunteers to choose between other types of objects, including food and magazines, produced similar results. The brain thus uses a general strategy for decision-making that saves time and effort, but which also introduces bias. The next step is to work out how downstream brain regions use the vmPFC signal to select the preferred option.
DOI: http://dx.doi.org/10.7554/eLife.20317.002
Introduction
Standard decision theory assumes that when faced with a choice, individuals first assign subjective values to each option, and then compare these values in order to select the best option ( Samuelson, 1938 ; Von Neumann and Morgenstern, 1947 ). Understanding the neural mechanisms governing this valuation/selection process has become a central aim in the field of decision neuroscience. A large set of fMRI evidence points to the ventro-medial Prefrontal Cortex (vmPFC) as a key player in the valuation process ( Bartra et al., 2013 ; Clithero and Rangel, 2014 ). Neural activity in the vmPFC reflects subjective values, either measured with likeability ratings or inferred from binary choices ( Kable and Glimcher, 2009 ; Rangel and Hare, 2010 ). In accordance with the idea of a common neural currency ( Levy and Glimcher, 2012 ), the vmPFC was found to encode the subjective value of many kinds of goods, such as food, money, trinkets, faces, paintings, charities, etc. ( Chib et al., 2009 ; Hare et al., 2010 ; Lebreton et al., 2009 ; Plassmann et al., 2007 ). Such value coding was observed not only during choice but also in the absence of choice, during passive viewing of items presented in the attentional focus or when performing a distractive task on these items ( Lebreton et al., 2009 ; Levy et al., 2011 ; Abitbol et al., 2015 ).
During binary choices, it has been repeatedly shown that vmPFC activity correlates with the relative value of the two options under consideration (V A –V B ). However, the framing of such decision value signal, i.e. what A and B actually represent, remains an unresolved issue. This question is of importance because the brain regions downstream in the decision process cannot operate the appropriate selection without knowing which option is favored by the relative value signal. In particular, the post-decisional frame that has often been reported ( Boorman et al., 2013 ; Hare et al., 2011 ; Hunt et al., 2012 ) provides a decision value signal between chosen and unchosen options (V ch -V unch ) that cannot be used for making the selection. A spatial frame, based on the location of options (e.g., V left -V right ), has been suggested but not supported by much experimental evidence regarding the vmPFC valuation signal ( Palminteri et al., 2009 ; Wunderlich et al., 2009 ; Skvortsova et al., 2014 ). A more promising suggestion is the attentional frame ( Krajbich et al., 2010 ), in which the decision value signal encoded in the vmPFC depends on which option is attended to (V att -V unatt ). Such framing provided a good account for vmPFC activity in a choice task where fixation patterns were imposed to subjects, and correctly predicted several features of spontaneous choice behavior by imposing a discount weight on the unattended option value ( Krajbich et al., 2010 ; Lim et al., 2011 ). Notably, the attentional frame predicts that more fixated options should be more frequently chosen, which might explain why vmPFC activity has been found to correlate with V ch -V unch in other studies. However, the attentional model assumes that visual exploration is random, which might be true in artificial laboratory tasks where subjects have no information about upcoming options, but not in natural situations where prior knowledge might play a role.
Here, we hypothesize that the framing of the decision value encoded in the vmPFC is imposed by prior preferences. In other words, vmPFC activity should scale positively with the value of the option that is preferred a priori, which we call the default option, and negatively with that of the alternative (V def -V alt ). This hypothesis is compatible with the observation that vmPFC activity correlates with V ch -V unch , since choices usually follows on prior preferences. Yet, the interpretation is fundamentally different, as V def -V alt is a pre-decisional value signal susceptible to drive option selection. Our hypothesis builds on the literature about optimal foraging, which argues that stay/switch choice is the natural case of decision-making ( Stephens and Krebs, 1986 ). In this framework, staying on a same patch is the default option against which all alternatives must be compared. Several studies investigated such stay/switch decisions and implicated the dorsal anterior cingulate cortex in promoting a shift away from the default option ( Hayden et al., 2011 ; Kolling et al., 2012 ; Kvitsiani et al., 2013 ), while others induced default policies by manipulating prior probabilities of being correct ( Boorman et al., 2013 ; Fleming et al., 2010 ; Mulder et al., 2012 ; Scheibe et al., 2010 ). Although experimental manipulations vary across these studies, the default option is always defined as the option that would be selected in the absence of further information processing about its value relative to the alternatives. This definition provides objective criteria to identify the default option in a choice set: it should be selected faster and more frequently than the alternatives.
Therefore, our hypothesis implies that prior preferences should (1) induce a bias in favor of the default option, and (2) determine the frame of the value comparison process. The purpose of the present study was to examine how these two constraints would shape the brain valuation signal. To do so, we exploited the hierarchical structure of preferences: individuals have global preferences between categories of goods that can be locally reversed when comparing particular items. For instance, someone may prefer pop to jazz music in general, but nonetheless pick Keith Jarrett if the only other option is Britney Spears. In a binary choice, the prior preference at the category level thus designates a default option (i.e., the item belonging to the preferred category), but the option values still need to be compared at the item level in order to reach a final decision.
We conducted an fMRI experiment where participants made binary choices between items belonging to different categories. Preferences between categories were inferred from likeability ratings that were collected for every item before the scanning session. In the following analyses, we first establish the presence of a bias toward the default option in both choice and response time, above and beyond the prior preference between categories. Using computational modeling, we provide evidence that the default bias is best accounted for by a shift in the starting point of a drift diffusion process, which is proportional to the prior preference between categories. Then, we show that the default bias is unrelated to gaze fixation pattern, precluding an attentional framing. Finally, we uncover two effects of prior preference in fMRI data: (1) vmPFC baseline activity reflects the a priori shift in favor of the default option, and (2) vmPFC evoked response represents the value of the default option, irrespective of the eventual choice.
Prior to the scanning session, participants (n = 24) rated the likeability of items belonging to three different domains (food, music, magazines). Each domain included four categories of 36 items (see Materials and methods). At that time, participants were unaware of these categories. This is because the presentation of items for likeability ratings was blocked by domain but not by categories, which were randomly intermixed. During the scanning session, subjects performed series of choices between two items ( Figure 1 ), knowing that one choice in each domain would be randomly selected at the end of the experiment and that they would stay in the lab for another 15 min to enjoy their reward (listening to the selected CD, eating the selected food and reading the selected magazine). Trials were blocked in a series of nine choices between items belonging to the same two categories within a same domain. The two categories were announced at the beginning of the block, such that subjects could form a prior preference (although they were not explicitly asked to do so). We quantified this prior preference as the difference between mean likeability ratings (across all items within each of the two categories), which is hereafter denoted as DV CAT . In most cases (84 ± 3% on average), preferences inferred from mean ratings matched the preferences between categories that subjects directly expressed in post-scanning debriefing tasks. Moreover, the confidence in these choices between categories, which subjects provided on an analog rating scale during debriefing, was significantly correlated to DV CAT (r = 0.44 ± 0.06, t(23) = 7.88, p = 5.10 −8 ). These explicit measures taken after the scanning session therefore validate our quantification of implicit preferences between categories. In the following, we analyze choices and response times to assess the presence of a bias in favor of the default option (i.e., the item belonging to the preferred category).

Figure 1. Choice task.
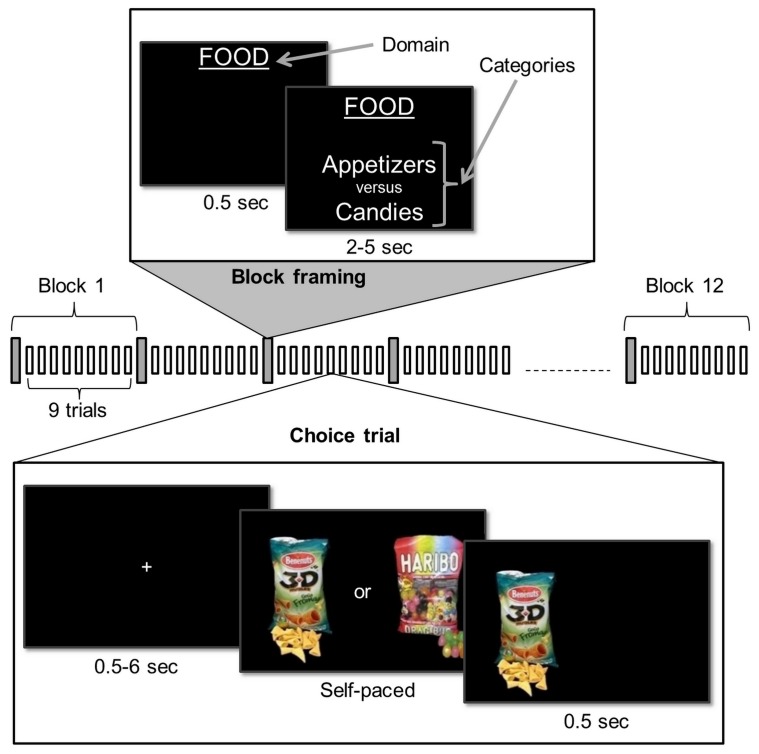
Participants performed the choice task inside the MRI scanner. The task was composed of four 12-block sessions. During a block, subjects first saw an instruction screen indicating the reward domain (e.g., food) and the two categories from which choice options were drawn. Then, they had to make a series of nine binary choices, each confronting the two categories with two novel items. The choice was self-paced and feedback on chosen option was provided to the subject.
DOI: http://dx.doi.org/10.7554/eLife.20317.003
We fitted a simple logistic regression model including a constant, the default option value, denoted V IT (def), and the alternative option value, denoted V IT (alt), to choices expressed in the ‘default vs. alternative’ frame. Regression coefficient estimates showed that the two option values were equally contributive to the choice (V IT (def): β = 0.060 ± 0.005, t(23) = 11.90, p = 3.10 −11 ; V IT (alt): β = −0.060 ± 0.004, t(23) = −14.21, p = 7.10 −13 ). Crucially, the constant was significantly positive (β = 0.68 ± 0.13, t(23) = 5.40, p = 2.10 −5 ), bringing evidence for a bias toward the default option. This constant was significantly reduced when including DV CAT in the regression model (β = 0.31 ± 0.16, t(23) = 1.94, p = 0.06), with the effect of DV CAT itself being significant (β = 0.021 ± 0.006, t(23) = 3.53, p = 2.10 −3 ), which established a direct link between prior preference and default bias. We also introduced past choices (coded 1 vs. −1 when default option was chosen vs. unchosen) in the regression model but they yielded no significant effect on choice rate. Consistently, the constant estimate was not different when restricting the logistic regression to the first choice in a block (β first = 0.58 ± 0.49, β all = 0.68 ± 0.13, difference: t(23) = 0.97 p = 0.44), confirming that the default bias was not resulting from the history of past choices. To illustrate this result ( Figure 2A ), we plotted the choice rate, P(def), as a function of the decision value, DV IT =V IT (def)-V IT (alt). This plot shows that even when the two options have the same value (DV IT = 0 on the x-axis), the choice rate is not at chance level (50% on the y-axis), which would denote indifference, but shifted toward the default option (by 15.7 ± 1.7% on average). Thus, these results provide behavioral evidence for a ‘choice bias’ occurring on top of the decision value (DV IT ), i.e. above and beyond what could be predicted by the difference in likeability rating.
Figure 2. Behavioral results (MRI experiment).
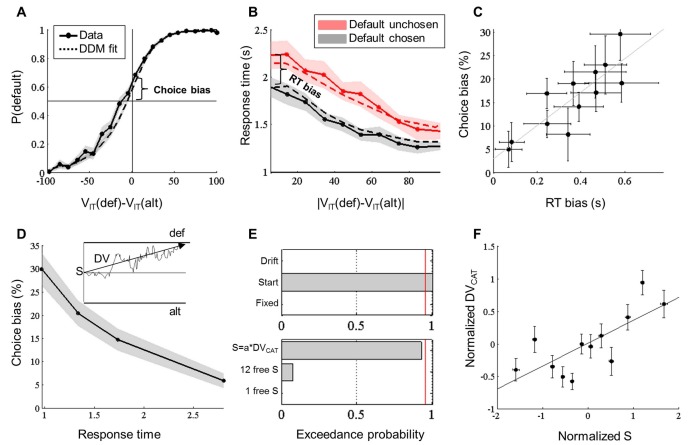
( A ) Probability of choosing the default option, P(def), plotted as a function of decision value, DV IT , divided into 20 bins. Values correspond to likeability ratings given by the subject prior to scanning. Both probabilities observed in choice data (solid line) and simulated from the fitted Drift Diffusion Model (dashed line) are shown. Choice bias was defined as the difference between the observed probability for a null decision value and the expected equiprobability (50%). ( B ) Choice response time (RT) plotted as a function of the absolute decision value, |DV IT |, divided into 10 bins, separately for trials in which the default option was chosen (black) and unchosen (red). Both RT observed in behavioral data (solid line) and simulated from the fitted Drift Diffusion Model (dashed line) are shown. RT bias was defined as the difference between the intercepts observed for the two types of choice. ( C ) Correlation of choice and RT biases across blocks. ( D ) Choice bias plotted as a function of response time, divided into four bins. Inset illustrates the Drift Diffusion model (adapted from ( Voss et al., 2013 ), with S the starting point, DV the mean drift rate and def / alt the thresholds for choosing default / alternative options. Choice bias was larger for shorter RT, suggesting that it could arise from a bias in the starting point. ( E ) Family model comparison between different theoretical accounts of choice and RT biases. Top: the null model (‘Fixed’) is compared to models in which either the starting point (‘Start’) or the drift rate (‘Drift’) is allowed to favor the default option. Bottom: the model with a single free starting point (‘1 free S’) is compared to models in which the starting point is varied across blocks, either in proportion to the value difference between categories ‘S=a*DV CAT ’ or as a set of 12 independent parameters (‘12 free S’). Red line corresponds to 95% exceedance probability. ( F ) Correlation across blocks between DV CAT and starting point S (from fitting the '12-free-S’ model). This suggests that the starting point is adjusted in each block to the average value difference between the two confronted categories. Shaded areas and error bars represent ± inter-subject SEM.
DOI: http://dx.doi.org/10.7554/eLife.20317.004
To account for choice response time (RT), we fitted a general linear model (GLM) including the main effects and the interaction of two factors: the unsigned decision value (|DV IT |) and the choice type (default vs. alternative). As typically reported, we found a significant effect of unsigned decision value (t(23) = −6.8, p = 6.10 −6 ), indicating that choices were longer when option values were closer. We also found a significant effect of choice type (t(23) = −5.47, p = 1.10 −5 ), indicating that subjects were faster to pick the default option than the alternative. There was no significant interaction between the two factors (t(23) = 0.59, p = 0.56). Thus the ‘RT bias’ corresponds to the difference between intercepts for a null decision value ( Figure 2B ). This RT bias means that subjects were significantly faster when choosing the default (by 357 ± 50 ms on average), irrespective of the decision value.
To assess whether the choice and RT biases could arise from the same underlying computation, we tested their correlation across blocks ( Figure 2C ). This is possible in our design because each block corresponds to a confrontation between two given categories, some being very close and others far apart in terms of mean likeability (i.e., they vary in terms of DV CAT ). We fitted a regression model to each block in order to extract choice and RT biases for each pair of categories. Correlation across blocks was estimated at the subject level and then tested against the null hypothesis at the group level. We found a significant correlation between the two biases (r = 0.24 ± 0.06, t(23) = 3.78, p = 1.10 −3 ), suggesting a common underlying mechanism, which we further characterized using computational modeling.
Computational modeling
To account for both choice and RT distributions, we employed an analytical approximation to the Drift Diffusion Model (DDM). The DDM assumes that choices result from a sequential sampling process, through which a decision variable accumulates evidence until it reaches a boundary ( Ratcliff, 1978 ; Ratcliff and McKoon, 2008 ). DDMs were originally developed to explain perceptual decisions but they have already been successfully applied to economic (value-based) decisions ( Gold and Shadlen, 2007 ; Basten et al., 2010 ; Krajbich et al., 2010 ; 2012 ). In our DDM, the boundaries corresponded to the default and alternative choices, and the mean of the drift rate was the signed decision value, DV IT (inset in Figure 2D ). A priori, the choice and RT biases could arise from a change in the drift rate or from a shift in the starting point, S. The latter possibility is more consistent with the negative correlation that was observed between choice bias and RT ( Figure 2D ) and tested at the group level (r = −0.68 ± 0.08, t(23) = −8.06, p = 4.10 −8 ). Indeed, in the DDM framework, a bias in the starting point has less impact on choices when the decision process lasts longer ( Brunton et al., 2013 ).
To formally disentangle between these possibilities, we compared a DDM where the starting point is fixed at zero and the drift rate equal to DV IT (null model) to six alternative DDMs where either the starting point or the drift rate is allowed to change across subjects, and for some of them across blocks. The first three models (‘start family’) test the hypothesis of a shift in the starting point. The shift was captured with a single free parameter in model 1 (‘1 free S’), with one free parameter per block in model 2 (‘12 free S’), or as a free parameter scaled by DV CAT in model 3 (‘S=a*DV CAT ’). Thus, the starting point was respectively considered constant across blocks (but possibly different from zero), freely adjusted to each block, or proportional to the prior preference. The last three models (drift family) test the hypothesis of a change in the drift rate, which in any case was proportional to DV IT . The change was captured with a single additional parameter to DV IT in model 4, with one additional parameter per block in model 5, and with an additional term scaled by DV CAT in model 6.
We first conducted a family model comparison to examine the possibilities that the choice and RT biases were due to a shift in the starting point (models 1–3) or a change in the drift rate (models 4–6), relative to the null model ( Figure 2E , top). The most plausible mechanism was the shift in the starting point (start family: exceedance probability, xp = 0.997). Then, we compared the three models within this family ( Figure 2E , bottom) and found evidence in favor of model 3 (xp = 0.920), suggesting that the starting point varied across blocks proportionally to prior preferences. We verified this conclusion by testing the correlation across blocks between the posterior means of the 12-free-S model and the prior preference DV CAT ( Figure 2F ). The correlation was significant at the group level (r = 0.35 ± 0.07, t(23) = 5.12, p = 3.10 −5 ), strengthening the idea that prior preference was imposing a shift in the starting point that resulted in both choice and RT biases. Thus, the correlation observed between choice and RT biases was driven by variations in DV CAT across blocks, the two biases trending to zero when DV CAT was close to null.
Eye-tracking
The fact that decision bias was best explained by shifting the starting point made less likely an interpretation in terms of attentional dynamics. This is because in previous studies, gaze fixation pattern was found to affect the drift rate and not the starting point ( Krajbich et al., 2010 ). We nevertheless investigated the possibility that the effect of prior preferences on choice and RT biases could be mediated by the pattern of gaze fixation. This possibility would imply that subjects pay more attention to the default option than to the alternative, which we examined using eye-tracking measurements.
Another group of participants (n = 23) performed the same series of rating and choice tasks, while their gaze position on the screen was recorded using an eye-tracking device. All the behavioral results described in the previous section were replicated ( Figure 3A and B ), with a significant bias in both choice (15.5 ± 1.7%, t(22) = 5.12, p = 4.10 −4 ) and RT (341 ± 42 ms, t(22) = −6.69, p = 1.10 −6 ), and a significant correlation between the two (r = 0.22 ± 0.06, t(22) = 3.87, p = 8.10 −4 ).
Figure 3. Behavioral results (eye-tracking experiment).
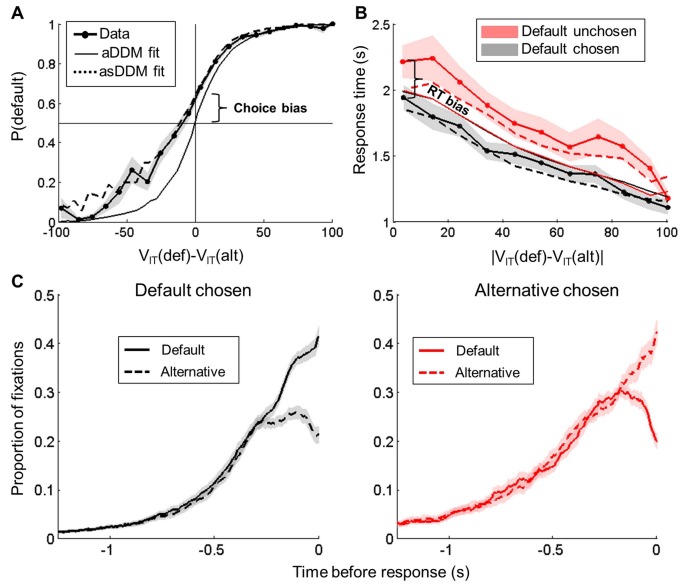
( A ) Probability of choosing the default option plotted as a function of decision value DV IT . The three curves correspond to probabilities actually observed in choice data (lines with circles) and simulated from either the fitted attentional Drift Diffusion Model (aDDM, solid lines) or the same model fitted with a starting point proportional to prior preference DV CAT (asDDM, dashed line). ( B ) Choice response time (RT) plotted as a function of the absolute decision value |DV IT |, separately for trials in which the default option was chosen (left) and unchosen (right). The different curves correspond to RT observed in behavioral data (lines with circles) and simulated from either the fitted aDDM (solid line with circles) or asDDM (dashed line). Note that the aDDM alone cannot reproduce choice and RT biases. ( C ) Proportion of fixations (number of trials over all trials) to the default and alternative options at each time point when default is chosen (left) or unchosen (right). Curves are time-locked to choice (button press). They do not add up to one because at a given time point in a given trial, subjects may fixate none of the two options. Shaded areas are ± inter-subject SEM.
DOI: http://dx.doi.org/10.7554/eLife.20317.005
We also replicated a number of results predicted by the attentional Drift Diffusion Model (aDDM), in which a parameter θ down-weights the value of the unattended item in the decision value, hence in the drift rate ( Krajbich et al., 2010 ; Krajbich and Rangel, 2011 ; Lim et al., 2011 ; Krajbich et al., 2012 ). As predicted by the aDDM, we notably observed that the choice probability was higher for the item fixated last (t(22) = −11.68, p = 7.10 −11 ), and for the most fixated item during the decision process (t(22) = −4.71, p = 1.10 −4 ), irrespective of decision value. These results confirm that fixation pattern had the expected effects on choice. However, none of these effects could account for the bias toward the default option that was observed in our task.
To test the link between prior preference and gaze fixation pattern, we compared the duration of fixation for the default and alternative options, separately for trials in which the default was chosen and unchosen. We found that the default option was fixated longer when it was chosen (difference: 81 ± 11 ms, t(22) = 7.52, p = 2.10 −7 ). Conversely, it was the alternative option that was fixated longer when the default option was not chosen (difference: 41 ± 17 ms, t(22) = 2.41, p = 2.10 −2 ). Consistently, the ANOVA conducted on fixation time revealed a significant main effect of the ‘chosen vs. unchosen’ factor (F(1,88) = 4.03, p = 0.048), but no main effect of the ‘default vs. alternative’ factor (F(1,88) = 0.43, p = 0.41). The interaction was significant (F(1,88) = 17.45, p = 1.10 −4 ), reflecting the fact that the default option was more frequently chosen, and with larger decision values. Thus, fixation duration was indeed predictive of choice, but was not influenced by prior preference. To control for the dynamics of the decision process, we computed the proportion of fixations for each option at each time point. The time courses locked to stimulus onset revealed a clear preference for looking at the left option during the first 250 ms, and then at the right option during the next 250 ms, but no significant preference for the default option at the beginning of the trial. The time courses locked on the response confirmed the fixation bias toward the chosen option, with a similar pattern whether default or alternative option was chosen ( Figure 3C ). This result was further confirmed by a model comparison showing that fixation duration for each option was better explained (xp = 0.999) by a GLM including the unsigned decision value and the choice (chosen vs. unchosen option) than by GLMs including an additional regressor that indicated the prior preference (default vs. alternative option).
Finally, we compared four variants of the DDM to contrast how fixation pattern and prior preference influence the decision process. The first was the null model, with a starting point S fixed at zero and a weighting factor θ fixed at one. The second was the sDDM selected as the best model in the first experiment, with S proportional to DV CAT and θ still fixed at one. The third was the standard aDDM, with S fixed at zero and a freely fitted θ. The fourth was termed asDDM and included both S proportional to DV CAT and fitted θ. The most plausible model was the asDDM (xp = 0.95), with the weight on DV CAT significantly above zero (0.02 ± 0.003, t(22) = 7.85, p = 1.10 −6 ), and a θ significantly below one (θ = 0.94 ± 0.03, t(22) = 2.14 p = 0.04). The fits of choice and RT are illustrated for the aDDM and asDDM ( Figure 3A and B ). Although using the fixation pattern (with θ) improved the fit, only the prior preference (with S) could explain the decision bias toward the default option. We reached similar conclusions when the advantage for the attended option was additively included in the drift rate, on top of decision value (as in Cavanagh et al., 2014 ). In fact, gaze fixation pattern failed to produce the default bias simply because the default option was no more looked at than the alternative option.
Our behavioral results establish that prior preferences exert a bias on choices, which in the DDM framework was best explained by a proportional shift in the starting point. We analyzed fMRI data first to examine whether the bias toward the default option could be observed in baseline neural activity, second to assess whether prior preference could frame the comparison between option values that might be implemented in the evoked neural response.
Baseline activity
To examine whether the prior preference (DV CAT ) was encoded in baseline activity, we fitted a GLM (GLM0, see Materials and methods) convolved with a finite impulse response (FIR) function to fMRI data. GLM0 contained an indicator delta function for option display that was parametrically modulated by the option values, V IT (def) and V IT (alt). In the following we analyze regression coefficient estimates obtained for the indicator function on volumes acquired before and after option display. The contrast performed at the individual level weighted block-specific indicator functions by z-scored DV CAT . Group-level statistical test (p<0.005, uncorrected) performed on this contrast for the volume acquired two seconds before option display revealed activity scaling with DV CAT in the vmPFC, ventral striatum and left hippocampus ( Figure 4A , left panel), which are regions classically identified as parts of the brain valuation system (e.g., Lebreton et al., 2009 ). The ventral striatum and left hippocampus were the only regions that survived cluster-level family-wise error (FWE) correction at the whole-brain level. The vmPFC cluster only survived small-volume correction (p = 6.10 −3 ) within an ROI based on independent criterion – a sphere centered on the peak of the cluster that positively reflected value in a previous meta-analysis ( Bartra et al., 2013 ). In order to illustrate the time course of this effect in the vmPFC, we simply averaged BOLD activity levels (coefficient estimates for indicator functions) in high and low DV CAT blocks separated with a median split ( Figure 4A , right panel). The difference between high and low DV CAT appeared to be maintained during the decision process and to progressively vanish at the end of the trial.
Figure 4. Neural correlates of the bias and framing effects of prior preference.
( A ) Bias in value coding and vmPFC baseline. Left: axial glass brain and sagittal slice of statistical maps relating to the prior preference (DV CAT ), one volume (2 s) before the display of choice options. Areas shown in black (on glass brain) and yellow (on sagittal slice) showed significant positive group-level random effect (one-sample t-test, p<0.005 uncorrected for display purposes, minimum extent: 100 voxels). Right: time course of peri-stimulus fMRI activity in the vmPFC region, shown separately for high (green) and low (purple) DV CAT . Black vertical line (time 0) indicates the onset of choice options. ( B ) Frame of value coding in vmPFC response. Left: axial glass brain and sagittal slice of statistical maps relating the canonical hemodynamic response to the decision value (DV IT ), at the onset of choice option (same threshold as in A: one-sample t-test, p<0.005 uncorrected for display purposes, minimum extent: 100 voxels). Right: time courses of the regression estimate (beta) obtained in the vmPFC region for the default option value when it was chosen (black) or unchosen (red). Shaded areas are ± inter-subject SEM.
DOI: http://dx.doi.org/10.7554/eLife.20317.006
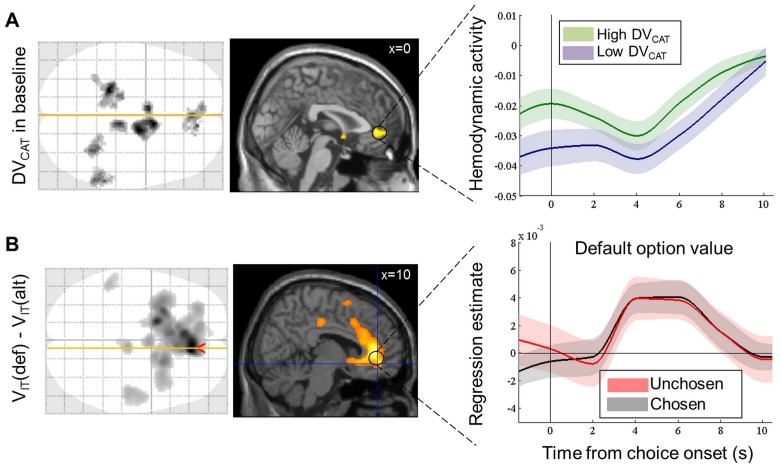
Figure 4—figure supplement 1. Dissociation of neural value representations expressed in the pre-choice versus post-choice frame.
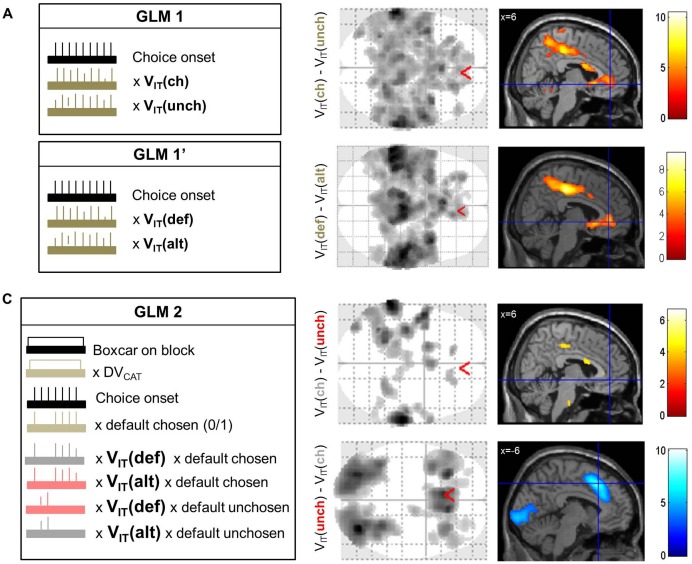
Evoked response
Our key hypothesis was that the decision value signal is framed by the prior preference, as a comparison between default and alternative options. This opposition of default vs. alternative options partially overlaps with that of chosen vs. unchosen options (here, in 77.8 ± 1.0% of the choices), the latter contrast being classically used to localize value comparison signals. We started the analysis by replicating this classical approach with a standard GLM, before dissociating the two possible frames with a more exhaustive GLM.
The first GLM only contained V IT (ch) and V IT (unch) as parametric modulators of a categorical regressor (delta function) indicating option display (GLM1, Figure 4—figure supplement 1A , left), all convolved with a canonical hemodynamic response function (HRF). As expected, the classical contrast V IT (ch)-V IT (unch) revealed significant correlation in brain valuation regions such as the vmPFC, ventral striatum and posterior cingulate cortex. Yet, this pattern of activation was not very specific of the brain valuation system, as it also included other brain regions such as the intra-parietal lobules. The opposite contrast, V IT (unch)-V IT (ch), yielded significant correlation in the dorsal anterior cingulate cortex (dACC) and bilateral anterior insula, which are classically associated with choice difficulty or with the value of foregone options ( Kolling et al., 2012 ; Shenhav et al., 2014 ), as well as in the inferior frontal gyrus and middle occipital gyrus. The equivalent GLM containing V IT (def) and V IT (alt) as parametric modulators yielded similar results (GLM1’, Figure 4—figure supplement 1A ), which is expected due to the shared variance between the chosen and default option values.
In order to disambiguate between representations of pre-choice values, V IT (def) and V IT (alt), and post-choice values, V IT (ch) and V IT (unch), we built a second GLM (GLM2, Figure 4—figure supplement 1B , left) that included two value regressors for each choice type (default chosen versus unchosen). These value regressors were parametric modulators of the categorical regressor indicating option display. The GLM also contained a regressor modeling the choice type itself, to dissociate value coding from option selection, and a boxcar function parametrically modulated by DV CAT , to account for tonic effects of prior preference. This GLM allows computing both the V IT (ch)-V IT (unch) and the V IT (def)-V IT (alt) contrasts on the canonical evoked response.
Critically, we found significant activation (surviving cluster-level, whole-brain FWE correction) in the vmPFC and ventral striatum with the V IT (def)-V IT (alt) contrast ( Figure 4B , left) but not with the V IT (ch)-V IT (unch) contrast ( Figure 4—figure supplement 1B , right). The neural response implementing the V IT (def)-V IT (alt) comparison was specific to the brain valuation system, since no other regions than vmPFC and ventral striatum passed the corrected threshold (Table S1 - Supplementary file 1 ). In particular, activity in the parietal or temporo-parietal cortex followed the V IT (ch)-V IT (unch) contrast as in the classical GLM. The opposite contrast, V IT (unch)-V IT (ch), again activated the dorsal anterior cingulate, anterior insula and middle occipital gyrus ( Figure 4—figure supplement 1B , right). The latter activation might relate to the fact that visual inspection of choice options was longer when the choice was more difficult. No brain region was significantly associated with the V IT (alt)-V IT (def) contrast.
As we realized that the evoked response might have contaminated baseline vmPFC activity at the next trial, we estimated another version of GLM2, with the four parametric modulators - V IT (def_chosen), V IT (alt_unchosen), V IT (def_unchosen), V IT (alt_chosen) – replaced by the same values but for the options presented in the previous trial (the first trial of each block was discarded). None of these parametric modulators had a significant effect at the time points preceding option display (t = −2 and t = 0 s from stimulus onset). Therefore, the values of the options presented in the previous trial did not affect baseline vmPFC activity, beyond the variance that they shared with DV CAT .
Thus, the neural decision value encoded in the vmPFC seemed to be expressed in the pre-choice frame (default minus alternative), rather than in the post-choice frame (chosen minus unchosen). However, inspecting the regression coefficient estimates obtained for V IT (def) and V IT (alt) separately suggested that the contrast was driven by V IT (def), as no significant effect was observed with V IT (alt) alone. To verify that V IT (def) was similarly encoded in the vmPFC irrespective of the eventual choice, we fitted a FIR version of GLM2, and extracted regression estimates from the same independent vmPFC ROI as used previously ( Bartra et al., 2013 ). We found that regression estimates for V IT (def) were significant for both choice types (default chosen: β = 6.10 −3 ± 2.10 −3 , t = 3.23, p = 3.10 −3 ; default unchosen: β = 6.10 −3 ± 3.10 −3 , t = 2.67, p = 0.01), four seconds after option display. For illustration purposes, we have plotted the time course of regression estimates extracted from the vmPFC cluster associated with V IT (def)-V IT (alt) in our main analysis ( Figure 4B , left).
To further challenge our conclusion regarding the encoding of decision value in the vmPFC, we compared variants of GLM2 that included different parametric regressors locked to option display. The two-by-two model space tested the possibilities of (1) pre-choice (default minus alternative) versus post-choice (chosen minus unchosen) framing for value coding and (2) best option value (V IT (def) or V IT (ch)) versus differential value coding (DV IT ). Bayesian model selection indicated that the pre-choice family was more plausible (xp = 0.92), and that within this family, V IT (def) alone better accounted for vmPFC activity than DV IT (xp = 0.94). By comparison, the post-choice family best accounted for dACC activity (xp = 0.99), with a clear advantage for the decision value DV IT over V IT (ch) alone (xp = 0.9996).
At first, we were surprised that the vmPFC (or any other brain region) seemed to hold no representation of V IT (alt) despite the fact that, behaviorally, V IT (alt) impacted choices to a similar extent as V IT (def). Then we reasoned that the vmPFC might have encoded both option values on top of the decision value, following on the idea that such valuation processes are automatically triggered when stimuli are presented ( Lebreton et al., 2009 ; Levy et al., 2011 ). This would imply that vmPFC activity should correlate not only with the difference between option values but also with their sum. The net result would be a correlation with V IT (def), since V IT (alt) would be subtracted out, which was observed in our analysis. In order to test this hypothesis, we fitted a last variant of GLM2 that included both the difference and sum of option values in the pre-choice frame as parametric modulators of option display. The common variance, linked to the presence of V IT (def) in both the sum and difference, was removed such that each regressor had unique variance. We examined the regression estimates extracted from the independent vmPFC ROI and found significant effects for both the difference (β def-alt = 0.10 ± 0.04, t(23) = 2.74, p = 0.01) and the sum (β def+alt = 0.14 ± 0.06, t(23) = 2.47, p = 0.02). In addition, model comparison showed that the GLM with orthogonalized sum and difference was a much better explanation of the vmPFC response (xp = 0.993) than the GLM containing only V IT (def). This result suggests that the vmPFC evoked response signals the two option values (sum) on top of the decision value (difference). In macroscopic measures of brain activity such as fMRI, the positive and negative correlation with V IT (alt) appear to cancel each other, but these representations might be dissociable using techniques with better spatial resolution that can access microscopic scales.
In this study, we examined how prior preference shapes the neural representation of decision value. We observed two major phenomena in vmPFC activity: (1) baseline activity was shifted in proportion to the strength of prior preference, as was the starting point in a drift diffusion model accounting for the decision bias in favor of the default option, (2) evoked activity signaled the value of the option belonging to the preferred category, suggesting that the choice was framed as a comparison between default and alternative.
Although they were not instructed to do so, subjects likely formed prior preferences at the beginning of blocks, when the two categories confronted in the upcoming series of choices were announced on the screen. Preference between the two categories was inferred from likeability ratings averaged across items belonging to each category. In a vast majority of cases, this notion of preference matched the preference directly expressed by the subjects in binary choices between categories made during post-scan debriefing. Moreover, the difference between mean likeability ratings (DV CAT ) was proportional to the confidence expressed in these choices between categories, in keeping with the notion that choice and confidence proceed from the same decision value ( De Martino et al., 2013 ). These debriefing observations validate our notion of prior preference, which then served to designate the default option in the pair of items that was presented for choice.
Indeed items from the preferred category could be qualified as default options, because they were chosen faster and more frequently than their alternatives. These choices and RT biases are minimal requirements for a default option, i.e. an option that should be chosen in the absence of further information processing. Such criteria have been used in other paradigms where the goal was to maximize an objective reward, with for instance the default being defined as the pre-selected option in a perceptual decision task ( Fleming et al., 2010 ), as the current patch in a foraging task ( Hayden et al., 2011 ; Kolling et al., 2012 ; Kvitsiani et al., 2013 ), or as the long-term best option in a probabilistic instrumental learning task ( Boorman et al., 2013 ). These studies reported that when the two option values were similar, subjects (both humans and monkeys) favored the default option. This phenomenon has been coined ‘default bias’, or ‘status quo bias’ in cases where the default option was defined as the pre-selected choice. Here, the same phenomenon was observed in the case of subjective preference. Importantly, the default bias was estimated once option values were matched, therefore it goes beyond what could be predicted from the difference in likeability ratings between categories. This bias could lead to preference reversals, meaning that subjects would pick the default option in spite of the alternative option having received a higher rating. Thus, the default bias denotes suboptimal decision-making, which could be compensated by the fact that following a default policy is on average less costly in terms of time or cognitive resource, than a systematic unbiased comparison of option values. This phenomenon is therefore much different from the optimal use of prior information that has been observed in a variety of perceptual decision-making paradigms, subjects being biased only when tricked with invalid cues ( Link and Heath, 1975 ; Bogacz et al., 2006 ; Scheibe et al., 2010 ; Mulder et al., 2012 ).
Within the drift diffusion framework, the default bias observed in choice and RT was best accounted for by a shift in the starting point. This is consistent with perceptual decision-making studies showing that prior information on probability or payoff is also incorporated in the starting point ( Scheibe et al., 2010 ; Summerfield and Koechlin, 2010 ; Mulder et al., 2012 ). However, this is not compatible with the idea that the effect of prior preference on choice and RT biases is mediated by the pattern of gaze fixations. This idea implies that subjects pay more attention to the default option, which through the attentional DDM mechanism would favor the default choice, because the attended option has more weight than the alternative in the drift rate. In fact, subjects looked equally often at the default and alternative options in the eye-tracking experiment. Our results nonetheless confirmed that the pattern of gaze fixation does inform the prediction of choices, in a manner that is nicely captured by the attentional DDM. Thus, although the attentional DDM is perfectly compatible with our data, it could not by itself explain the default bias. The best account of choice and RT was in fact obtained with a model that cumulated the down-weighting of unattended options in the drift rate, as suggested by the attentional DDM, and the shift in the starting point that explains the default bias.
In our best model, the shift in starting point was proportional to the prior preference (DV CAT ). A striking parallel was found at the neural level, with the prior preference being reflected in the baseline activity of valuation regions including the vmPFC, ventral striatum and posterior cingulate cortex. This is in line with a previous study showing that baseline vmPFC activity is sensitive to contextual factors, both in humans and monkeys, and provide a bias in subsequent valuation processes ( Abitbol et al., 2015 ). Other contextual manipulations were found to modulate vmPFC activity and subsequent valuation, for instance mood induction has been shown to affect reward-related vmPFC activity ( Young and Nusslock, 2016 ). In contrast, cueing manipulation that affected perceptual decisions through a shift in starting point had no influence on vmPFC activity ( Scheibe et al., 2010 ; Summerfield and Koechlin, 2010 ; Mulder et al., 2012 ). This dissociation suggests that the recruitment of vmPFC was not related to the general process of changing the accumulation starting point, but to biasing value-based decisions (as opposed to perceptual decisions). In fact the shift in baseline vmPFC activity was maintained throughout the decision process, and was hence added to the evoked activity, which followed a canonical hemodynamic response. As both baseline and evoked activity scaled with the value of the default, respectively at the category and item levels, they together contributed to favoring the default option over the alternative. Thus, the mechanics is analog to the DDM process, but the dynamics is somewhat different. In fact, the neural dynamics is not compatible with the vmPFC implementing the DDM, since we observed no ramping signal corresponding to an accumulation-to-bound process; neither is it compatible with the vmPFC output being sent to a distant accumulator, since the shift in starting point should not be integrated over time. Therefore, we do not suggest that the DDM used to capture behavioral patterns is literally implemented as such in the brain, just that the general logics and some key features appeared to match vmPFC activity during choices. We also note that other types of modeling would have been possible to capture behavioral effects, notably a Bayesian account where prior preference would affect the mean and perhaps the variance of a prior distribution on decision value.
The analysis of the evoked response showed that the vmPFC and ventral striatum encode the decision value in a frame that opposes the default to the alternative option. This pre-choice framing supports the idea of an anatomical separation between the valuation and selection processes, with the vmPFC being implicated in the former but not the latter. It could be a very general frame for value coding in the vmPFC, because most studies found a correlation between vmPFC activity and the value of chosen options (e.g., Hare et al., 2011 ; Boorman et al., 2013 ), which are partially confounded with default options as we have shown here. We note that an opposite dissociation was found by Boorman et al. (2013) , with the vmPFC encoding option values in post-choice frame, and not pre-choice frame. As decision-making dynamics was not explored in this study, it is unclear whether participants truly implemented a default strategy as defined here, which implies an anticipation of a default response, associated with shortening of response time. Accordingly, the representation of chosen option value was largely delayed in comparison to our study (peaking 10 s after option display), possibly related to the necessity of storing expected values in a learning context.
Another partial confound is with choice easiness or confidence, which was also found to be integrated in vmPFC activity in addition to value ( De Martino et al., 2013 ; Lebreton et al., 2015 ). The pre-choice framing could also be reconciled with the theory that the vmPFC encodes the value of the attended option, if we assume that when they have no prior information on the choice, subjects set up a default on the fly, which could be the option they just look at. By contrast, we found a post-choice framing of decision value (unchosen vs. chosen) in the dACC and anterior insula, which could be related either to choice difficulty or to the value of shifting away from the default policy, which might require cognitive control ( Hare et al., 2011 ; Kolling et al., 2012 ; Shenhav et al., 2013 ).
A last potential issue is that the correlation with decision value (DV IT ) was driven by the default option, although the default and alternative options had the same weight on choices, and despite the two options being reflected in other regions such as dACC. Our interpretation is that both option values are encoded in the vmPFC on top of the decision value. As a result, the correlation with the alternative option value would be cancelled out, and the correlation with the default option value would be doubled, as suggested by the following equation:
This interpretation is consistent with both the idea that the vmPFC automatically encodes the value of items that fall under the attentional focus ( Lebreton et al., 2009 ; Levy et al., 2011 ) and the idea that the vmPFC computes a decision value whenever a choice process is engaged ( Plassmann et al., 2007 ; Grueschow et al., 2015 ). It would also explain why many studies report a correlation with the chosen value alone and not the decision value, as the unchosen value would be cancelled out for the same reasons ( Wunderlich et al., 2010 ; Kolling et al., 2012 ; Hunt et al., 2012 ). Model comparison supported this post-hoc interpretation, as including the two option values (sum) on top of the decision value (difference) provided the best account of vmPFC activity during choice. Other techniques than fMRI, with better spatial resolution, would be needed to investigate whether the different value representations rely on different populations on neurons.
In conclusion, our findings show that when decision-makers have a prior preference, the brain valuation system is configured so as to compare default and alternative options, with prior and novel information being encoded in baseline and evoked activity, respectively. Such framing could have been selected to solve natural decision problems, with the advantage of saving time and/or cognitive resource, and the disadvantage of biasing choice toward the default policy. How the valuation system adapts to artificial economic choices, in which two novel options present themselves simultaneously, still needs to be investigated. One may speculate that the brain would start by defining a default option, and then proceed to the comparison as usual. If this is correct, identifying the trial-wise and/or subject-wise default policy might be essential for understanding how the brain makes value-based decisions. However, we only have a proof of concept here, the generality of the ‘default vs. alternative framing’ remains to be established. Further research is also required to specify the contribution of the different brain regions that are involved in the valuation and selection processes, notably the dACC. The present results suggest that the vmPFC provides a decision value, which is also represented in the ventral striatum. How such decision value is used by the brain to make a selection remains to be explained.
Materials and methods
Participants.
The study was approved by the Pitié-Salpétrière Hospital ethics committee. All subjects were recruited via e-mail within an academic database and gave informed consent before participation in the study. They were right-handed, between 20 and 32 years old, with normal vision, no history of neurological or psychiatric disease, and no contra-indication to MRI (pregnancy, claustrophobia, metallic implants). They were not informed during recruitment that they would win food items, music CD and magazines to avoid biasing the sample. In total, 24 subjects (23.8 ± 2.8 years old, 12 females) were included in the fMRI experiment and paid a fixed amount (80€) for their participation. In the eye-tracking experiment, 24 right-handed subjects (24 ± 3.4 years old, 11 females) were recruited following the same procedure with the same inclusion criteria. No statistical method was used to predetermine sample size, but our sample size is similar to those generally employed in the field. One subject was excluded due to a technical issue with the eye-tracking device.
All tasks were programmed on a PC in MATLAB language, using the Psychophysics Toolbox extensions (RRID: SCR_002881 , Brainard, 1997 ; Pelli, 1997 ). Subjects performed the rating task outside the scanner and the choice task during fMRI scanning. Prior to each task, they were instructed and trained on short versions (24 trials) to get familiarized with the range of items and the mode of response.
During the rating task, subjects were asked to estimate the likeability of all 432 items that they could potentially obtain at the end of the experiment. These items were blocked by reward domain: food, music and magazines. Unbeknown to subjects, each reward domain was divided into 4 categories of 36 items. The 12 categories were: appetizers, biscuits, candies, chocolate (food domain); news, comics, cultural, generalist (magazine domain); French, jazz, rock, urban (music domain). The order of presentation was randomized within each reward domain, such that the categories were intermingled. The series of trials consisted of displaying pictures of the items one by one on the computer screen. A pseudo-continuous rating scale (101 points) was presented below the picture, with three reference graduations (do not like at all, neutral, like a lot). Subjects could move a cursor along the scale by pressing a key with the right index finger to go left or another key with the right middle finger to go right. The cursor was initially positioned at the middle of the rating scale. The rating was self-paced and subjects had to press a button with the left hand to validate their response and proceed to the next trial. At the beginning of each block, the reward domain was announced on a black screen.
Likeability ratings were used for pairing options in the choice task. For each domain, mean ratings were used to rank categories according to subjective preference. The most preferred categories (ranked 1 and 2) were opposed to the least preferred ones (ranked 3 and 4), making a total of 4 oppositions (1–3, 1–4, 2–3, 2–4). To generate the series of choices for each opposition, items were sorted in the order of likeability rating. Half the choices varied the difference between ratings while keeping the average constant (item ranked mean+X was paired with item ranked mean-X); the other half varied the average while keeping the difference minimal (item ranked X was paired with item ranked X-1). Thus, the mean value and relative value of choice options were orthogonalized. A total of 36 choices were generated for each inter-categorical opposition, and presented in a randomized order. The 36 choices were divided into 4 blocks of 9 trials, presented in 4 different fMRI sessions. As there were 12 possible oppositions (4 per domain), this makes a total of 432 trials, meaning that each item being presented twice.
At the beginning of each block, the domain was announced on a black screen for 0.5 s, then the two opposed categories were displayed for 2 to 5 s, followed by a 0.5 s fixation cross. Each block was composed of a series of 9 choices. Choice trials started with the display of the two options side by side. The side of a given category as well as the best rated option was counter-balanced across trials. Subjects were asked to indicate their preference by pressing one of two buttons, with their left or right index finger, corresponding to the left and right options. The chosen picture was framed with a white square for 0.5 s, followed by a black screen with fixation cross lasting for 0.5 to 6 s.
Importantly, subjects were not asked to generate a prior preference at the beginning of blocks, when the categories are revealed. They were only told that contextual information would be given before each series of choices, and that it would not require any response from their part. They also knew that at the end of the experiment, one trial per domain would be randomly selected and that they would be given the options chosen in these trials.
Following the scanning session, subjects had to complete a debriefing task in which they were presented the opposed categories two by two. They were asked to first select the category that they preferred and then to rate their confidence in their choice using an analog scale. Finally, they spent an additional 20 min in the lab to eat the food item, listen to the music album and read the magazine that they just won.
All analyses were performed with Matlab Statistical Toolbox (Matlab R20013b, The MathWorks, Inc., USA). Two dependent variables were recorded: choice (which option was selected) and response time (between option onset and button press). The influence of likeability ratings on these variables was assessed with logistic or linear regression models, as explained in the results section. Regression estimates were computed at the individual level and tested for significance at the group level using one-sample two-tailed t-test. Correlations between variables of interest were also computed at the individual level, using Pearson’s coefficient, and similarly tested at the group level.
In the eye-tracking experiment, gaze position was recorded with a 60 Hz sampling frequency using The Eye Tribe device ( http://theeyetribe.com ), during each block of the choice task. There was no constraint on the head, subjects were simply asked to avoid head movement. A screen providing feedback on the eye position was inserted in the trial sequence every time gaze was lost. The number of excluded trials due to loss of gaze position varied between 0 and 6, depending on subjects.
Fixation duration was computed for each trial and option, as the time during which gaze position was inside a square window delineated the corresponding picture on the screen. Last fixation was defined as the picture being looked at when the choice was made. The proportion of fixation was calculated as the number of trials in which gaze position was on the corresponding picture at a given time point. Note that these proportions for the two options do not add up to one because the gaze position can be outside the two windows.
We used the EZ2 analytical approximation for the drift diffusion model ( Wagenmakers et al., 2007 ; Grasman et al., 2009 ) to account for the probability of choosing the default option and the response time, on a trial-by-trial basis. As proposed by ( Ratcliff, 1978 ; Wagenmakers et al., 2007 ; Grasman et al., 2009 ), we defined the probability of choosing the default option as:
As proposed by EZ2 ( Grasman et al., 2009 ), we defined the corresponding RT as:
With φ ( x , y ) = e − 2 μ y σ 2 − e − 2 μ x σ 2 , A the amplitude between boundaries, S the starting point, μ the mean of the drift rate, σ the standard deviation of the drift rate and T n d the non-decision time. To compute response time in trials where the alternative option is chosen, we replace ( μ , S ) by ( − μ , A − S ) .
The free parameters A , T n d , σ , μ and S were estimated with the behavioral data. Different versions of the model were compared to account for the changes in choice and RT patterns that were induced across blocks by the variations in prior preference. In all cases, A , T n d and σ , were estimated for each individual but constant across blocks. In the null model, μ was proportional to the decision value (difference in likeability rating between options, DV IT , such that μ=αDV IT ) and S was set to zero. The model space (see details in the results section) explored the possibilities that μ and S could differ from their initial setting (μ=αDV IT + β / S=z), vary across blocks (12 free α for μ/12 free z for S), or be informed by the prior preference (difference in mean likeability rating between categories, DV CAT , such that μ=αDV IT + βDV CAT / S= βDV CAT ). In the attentional versions of the model, μ was also informed by gaze fixations, as follows:
With D d e f and D a l t the total durations of fixation for the default and the alternative options during the considered trial, and θ the weight discounting the value of the unfixated item relative to the fixated one ( Krajbich et al., 2010 ).
All versions of the drift diffusion model were fitted separately for each individual to choices and RTs using Matlab VBA-toolbox (available at http://mbb-team.github.io/VBA-toolbox/ ), which implements Variational Bayesian analysis under the Laplace approximation ( Daunizeau et al., 2014 ). This iterative algorithm provides a free-energy approximation for the model evidence, which represents a natural trade-off between model accuracy (goodness of fit) and complexity (degrees of freedom) ( Friston et al., 2007 ; Penny, 2012 ). Additionally the algorithm provides an estimate of the posterior density over the model free parameters, starting with Gaussian priors. Individual log model evidences were then taken to group-level random-effect Bayesian model selection (BMS) procedure ( Penny et al., 2010 ). BMS provide an exceedance probability (xp) that measures how likely it is that a given model (or family of models) is more frequently implemented, relative to all the others considered in the model space, in the population from which participants were drawn ( Rigoux et al., 2014 ; Stephan et al., 2009 ).
Functional echo-planar images (EPIs) were acquired with a T2*-weighted contrast on a 3 T magnetic resonance scanner (Siemens Trio). Interleaved 2 mm slices separated by a 1.5 mm gap and oriented along a 30° tilted plane were acquired to cover the whole brain with a repetition time of 2.01 s. The first five scans were discarded to allow for equilibration effects. All analyses were performed using statistical parametric mapping (SPM8, RRID: SCR_007037 ) environment (Wellcome Trust Center for NeuroImaging, London, UK). Structural T1-weighted images were coregistered with the mean EPI, segmented, and normalized to the standard Montreal Neurological Institute (MNI) T1 template. Normalized T1-images were averaged across subjects to localize group-level functional activations by superimposition. During preprocessing, EPIs were spatially realigned, normalized (using the same transformation as for structural images), and smoothed with an 8 mm full-width at half-maximum Gaussian kernel.
We used four general linear models (GLMs) to explain pre-processed time-series at the individual level.
The first model (GLM0) was built for whole-brain search of voxels encoding prior preference in baseline activity. It was composed of a finite impulse response function (FIR) that included seven time points per trial, from one TR (−2.01 s) before to five TR (10.05 s) after choice onset. The different blocks were modeled in separate regressors, each being parametrically modulated by the two option values (default and alternative). For each time point, we computed a contrast that weighted all trials of a given block by the corresponding prior preference (DV CAT ). Four subjects were excluded from this analysis because they presented at least one block without a sufficient variance to estimate the parametric regression coefficients.
The second model (GLM1) included a stick function capturing option display (only one event per trial), parametrically modulated by the two option values (chosen and unchosen). The three regressors were convolved with a canonical hemodynamic response function.
The third model (GLM2) included two categorical regressors: a boxcar function over blocks and the same stick function as in GLM1. The boxcar function was parametrically modulated by DV CAT , to account for tonic effects of prior preference. The stick function was parametrically modulated by five variables: chosen option (default or alternative), V IT (def) when default chosen, V IT (alt) when default chosen, V IT (def) when default unchosen and V IT (alt) when default unchosen. This allowed computing orthogonal contrasts for the decision value in the pre-choice (default vs. alternative) and post-choice (chosen vs. unchosen) frames. The regressors were convolved with a canonical HRF to localize brain regions where the evoked response reflected the decision value. In a subsequent analysis the same regressors were convolved with the same FIR as used for GLM0, in order to examine the dynamics of value coding in regions of interest (ROI).
The fourth model (GLM3) was equivalent to GLM1 except that the stick function modeling option display was modulated by the sum and difference of option values, in the pre-choice frame (default vs. alternative). Common variance between the two parametric regressors was removed such that they could explain a unique variance in the BOLD signal.
Motion artifacts were corrected in all GLMs by adding subject-specific realignment parameters as covariates of no interest. Regression coefficients were estimated at the individual level and then taken to group-level random-effect analysis using one-sample two-tailed t-test. In ROI analyses they were extracted from spheres of 6 mm radius positioned on coordinates defined independently from the present dataset: for the vmPFC we took the peak coordinates [−2 40 –8] from a meta-analysis of value representation ( Bartra et al., 2013 ), and for the dACC we took the peak coordinate [−6 24 34] of a negative correlation with chosen option value ( Boorman et al., 2013 ).
Four variants of GLM2 were also compared to better characterize value coding in the two ROI. The four regressors modeling option values were replaced by a single regressor: (1) default option value, (2) pre-choice decision value (default minus alternative), (3) chosen option value, (4) post-choice decision value (chosen minus unchosen). This was meant to assess whether value representation concerned only one option or the difference between the two, and whether it was expressed in a pre-choice or post-choice frame. All models were fitted to individual time-series extracted from vmPFC and dACC ROI, so as to compute group-level exceedance probabilities, following a BMS procedure similar to that used for behavioral data analysis.
Acknowledgements
We are grateful to the PRISME platform for help in behavioral and eye-tracking data collection and to the CENIR staff for assistance in MRI data acquisition. The study was funded by a Starting Grant for the European Research Council (ERC-BioMotiv). This work also benefited from the program 'Investissements d’avenir' (ANR-10-IAIHU-06). AL-P received a PhD fellowship from the Direction Générale de l’Armement and from the LabEx Bio-Psy. The funders had no role in study design, data collection and analysis, decision to publish or preparation of the manuscript. We wish to thank Antonio Rangel for suggestions on data analysis and Lionel Rigoux for guidance on computational modeling.
Funding Statement
The funders had no role in study design, data collection and interpretation, or the decision to submit the work for publication.
Funding Information
This paper was supported by the following grants:
Direction Générale de l’Armement to Alizée Lopez-Persem.
LabEx BioPsy to Alizée Lopez-Persem.
European Research Council ERC-BioMotiv to Mathias Pessiglione.
Agence Nationale de la Recherche ANR-10-9AIHU-06 to Mathias Pessiglione.
Additional information
Competing interests.
The authors declare that no competing interests exist.
Author contributions
AL-P, Conception and design, Acquisition of data, Analysis and interpretation of data, Drafting or revising the article.
PD, Conception and design, Analysis and interpretation of data, Drafting or revising the article.
MP, Conception and design, Analysis and interpretation of data, Drafting or revising the article.
Human subjects: The study was approved by the Pitie-Salpetriere Hospital ethics committee (protocole C12-69). All subjects were recruited via e-mail within an academic database and gave informed consent to participate and consent to publish before participation in the study.
Additional files
Regions are listed that survived voxel-based thresholding of p<0.001 uncorrected, and whole-brain 991 cluster-level FWE correction. [x, y, z] coordinates refer to the Montreal Neurological Institute (MNI) space.
DOI: http://dx.doi.org/10.7554/eLife.20317.008
- Abitbol R, Lebreton M, Hollard G, Richmond BJ, Bouret S, Pessiglione M. Neural mechanisms underlying contextual dependency of subjective values: converging evidence from monkeys and humans. Journal of Neuroscience. 2015;35:2308–2320. doi: 10.1523/JNEUROSCI.1878-14.2015. [ DOI ] [ PMC free article ] [ PubMed ] [ Google Scholar ]
- Bartra O, McGuire JT, Kable JW. The valuation system: a coordinate-based meta-analysis of BOLD fMRI experiments examining neural correlates of subjective value. NeuroImage. 2013;76:412–427. doi: 10.1016/j.neuroimage.2013.02.063. [ DOI ] [ PMC free article ] [ PubMed ] [ Google Scholar ]
- Basten U, Biele G, Heekeren HR, Fiebach CJ. How the brain integrates costs and benefits during decision making. PNAS. 2010;107:21767–21772. doi: 10.1073/pnas.0908104107. [ DOI ] [ PMC free article ] [ PubMed ] [ Google Scholar ]
- Bogacz R, Brown E, Moehlis J, Holmes P, Cohen JD. The physics of optimal decision making: a formal analysis of models of performance in two-alternative forced-choice tasks. Psychological Review. 2006;113:700–765. doi: 10.1037/0033-295X.113.4.700. [ DOI ] [ PubMed ] [ Google Scholar ]
- Boorman ED, Rushworth MF, Behrens TE. Ventromedial prefrontal and anterior cingulate cortex adopt choice and default reference frames during sequential multi-alternative choice. Journal of Neuroscience. 2013;33:2242–2253. doi: 10.1523/JNEUROSCI.3022-12.2013. [ DOI ] [ PMC free article ] [ PubMed ] [ Google Scholar ]
- Brainard DH. The psychophysics toolbox. Spatial Vision. 1997;10:433–436. doi: 10.1163/156856897X00357. [ DOI ] [ PubMed ] [ Google Scholar ]
- Brunton BW, Botvinick MM, Brody CD. Rats and humans can optimally accumulate evidence for decision-making. Science. 2013;340:95–98. doi: 10.1126/science.1233912. [ DOI ] [ PubMed ] [ Google Scholar ]
- Cavanagh JF, Wiecki TV, Kochar A, Frank MJ. Eye tracking and pupillometry are indicators of dissociable latent decision processes. Journal of Experimental Psychology: General. 2014;143:1476–1488. doi: 10.1037/a0035813. [ DOI ] [ PMC free article ] [ PubMed ] [ Google Scholar ]
- Chib VS, Rangel A, Shimojo S, O'Doherty JP. Evidence for a common representation of decision values for dissimilar goods in human ventromedial prefrontal cortex. Journal of Neuroscience. 2009;29:12315–12320. doi: 10.1523/JNEUROSCI.2575-09.2009. [ DOI ] [ PMC free article ] [ PubMed ] [ Google Scholar ]
- Clithero JA, Rangel A. Informatic parcellation of the network involved in the computation of subjective value. Social Cognitive and Affective Neuroscience. 2014;9:1289–1302. doi: 10.1093/scan/nst106. [ DOI ] [ PMC free article ] [ PubMed ] [ Google Scholar ]
- Daunizeau J, Adam V, Rigoux L. VBA: a probabilistic treatment of nonlinear models for neurobiological and behavioural data. PLoS Computational Biology. 2014;10:e1003441. doi: 10.1371/journal.pcbi.1003441. [ DOI ] [ PMC free article ] [ PubMed ] [ Google Scholar ]
- De Martino B, Fleming SM, Garrett N, Dolan RJ. Confidence in value-based choice. Nature Neuroscience. 2013;16:105–110. doi: 10.1038/nn.3279. [ DOI ] [ PMC free article ] [ PubMed ] [ Google Scholar ]
- Fleming SM, Thomas CL, Dolan RJ. Overcoming status quo bias in the human brain. PNAS. 2010;107:6005–6009. doi: 10.1073/pnas.0910380107. [ DOI ] [ PMC free article ] [ PubMed ] [ Google Scholar ]
- Friston K, Mattout J, Trujillo-Barreto N, Ashburner J, Penny W. Variational free energy and the Laplace approximation. NeuroImage. 2007;34:220–234. doi: 10.1016/j.neuroimage.2006.08.035. [ DOI ] [ PubMed ] [ Google Scholar ]
- Gold JI, Shadlen MN. The neural basis of decision making. Annual Review of Neuroscience. 2007;30:535–574. doi: 10.1146/annurev.neuro.29.051605.113038. [ DOI ] [ PubMed ] [ Google Scholar ]
- Grasman RPPP, Wagenmakers E-J, van der Maas HLJ. On the mean and variance of response times under the diffusion model with an application to parameter estimation. Journal of Mathematical Psychology. 2009;53:55–68. doi: 10.1016/j.jmp.2009.01.006. [ DOI ] [ Google Scholar ]
- Grueschow M, Polania R, Hare TA, Ruff CC. Automatic versus choice-dependent value representations in the human brain. Neuron. 2015;85:874 –87885. doi: 10.1016/j.neuron.2014.12.054. [ DOI ] [ PubMed ] [ Google Scholar ]
- Hare TA, Camerer CF, Knoepfle DT, Rangel A. Value computations in ventral medial prefrontal cortex during charitable decision making incorporate input from regions involved in social cognition. Journal of Neuroscience. 2010;30:583–590. doi: 10.1523/JNEUROSCI.4089-09.2010. [ DOI ] [ PMC free article ] [ PubMed ] [ Google Scholar ]
- Hare TA, Schultz W, Camerer CF, O'Doherty JP, Rangel A. Transformation of stimulus value signals into motor commands during simple choice. PNAS. 2011;108:18120–18125. doi: 10.1073/pnas.1109322108. [ DOI ] [ PMC free article ] [ PubMed ] [ Google Scholar ]
- Hayden BY, Pearson JM, Platt ML. Neuronal basis of sequential foraging decisions in a patchy environment. Nature Neuroscience. 2011;14:933–939. doi: 10.1038/nn.2856. [ DOI ] [ PMC free article ] [ PubMed ] [ Google Scholar ]
- Hunt LT, Kolling N, Soltani A, Woolrich MW, Rushworth MF, Behrens TE. Mechanisms underlying cortical activity during value-guided choice. Nature Neuroscience. 2012;15:470–S3. doi: 10.1038/nn.3017. [ DOI ] [ PMC free article ] [ PubMed ] [ Google Scholar ]
- Kable JW, Glimcher PW. The neurobiology of decision: consensus and controversy. Neuron. 2009;63:733–745. doi: 10.1016/j.neuron.2009.09.003. [ DOI ] [ PMC free article ] [ PubMed ] [ Google Scholar ]
- Kolling N, Behrens TE, Mars RB, Rushworth MF. Neural mechanisms of foraging. Science. 2012;336:95–98. doi: 10.1126/science.1216930. [ DOI ] [ PMC free article ] [ PubMed ] [ Google Scholar ]
- Krajbich I, Armel C, Rangel A. Visual fixations and the computation and comparison of value in simple choice. Nature Neuroscience. 2010;13:1292–1298. doi: 10.1038/nn.2635. [ DOI ] [ PubMed ] [ Google Scholar ]
- Krajbich I, Lu D, Camerer C, Rangel A. The attentional drift-diffusion model extends to simple purchasing decisions. Frontiers in Psychology. 2012;3:193. doi: 10.3389/fpsyg.2012.00193. [ DOI ] [ PMC free article ] [ PubMed ] [ Google Scholar ]
- Krajbich I, Rangel A. Multialternative drift-diffusion model predicts the relationship between visual fixations and choice in value-based decisions. PNAS. 2011;108:13852–13857. doi: 10.1073/pnas.1101328108. [ DOI ] [ PMC free article ] [ PubMed ] [ Google Scholar ]
- Kvitsiani D, Ranade S, Hangya B, Taniguchi H, Huang JZ, Kepecs A. Distinct behavioural and network correlates of two interneuron types in prefrontal cortex. Nature. 2013;498:363–366. doi: 10.1038/nature12176. [ DOI ] [ PMC free article ] [ PubMed ] [ Google Scholar ]
- Lebreton M, Abitbol R, Daunizeau J, Pessiglione M. Automatic integration of confidence in the brain valuation signal. Nature Neuroscience. 2015;18:1159–1167. doi: 10.1038/nn.4064. [ DOI ] [ PubMed ] [ Google Scholar ]
- Lebreton M, Jorge S, Michel V, Thirion B, Pessiglione M. An automatic valuation system in the human brain: evidence from functional neuroimaging. Neuron. 2009;64:431–439. doi: 10.1016/j.neuron.2009.09.040. [ DOI ] [ PubMed ] [ Google Scholar ]
- Levy DJ, Glimcher PW. The root of all value: a neural common currency for choice. Current Opinion in Neurobiology. 2012;22:1027 –1038. doi: 10.1016/j.conb.2012.06.001. [ DOI ] [ PMC free article ] [ PubMed ] [ Google Scholar ]
- Levy I, Lazzaro SC, Rutledge RB, Glimcher PW. Choice from non-choice: predicting consumer preferences from blood oxygenation level-dependent signals obtained during passive viewing. Journal of Neuroscience. 2011;31:118–125. doi: 10.1523/JNEUROSCI.3214-10.2011. [ DOI ] [ PMC free article ] [ PubMed ] [ Google Scholar ]
- Lim SL, O'Doherty JP, Rangel A. The decision value computations in the vmPFC and striatum use a relative value code that is guided by visual attention. Journal of Neuroscience. 2011;31:13214–13223. doi: 10.1523/JNEUROSCI.1246-11.2011. [ DOI ] [ PMC free article ] [ PubMed ] [ Google Scholar ]
- Link SW, Heath RA. A sequential theory of psychological discrimination. Psychometrika. 1975;40:77–105. doi: 10.1007/BF02291481. [ DOI ] [ Google Scholar ]
- Mulder MJ, Wagenmakers EJ, Ratcliff R, Boekel W, Forstmann BU. Bias in the brain: a diffusion model analysis of prior probability and potential payoff. Journal of Neuroscience. 2012;32:2335–2343. doi: 10.1523/JNEUROSCI.4156-11.2012. [ DOI ] [ PMC free article ] [ PubMed ] [ Google Scholar ]
- Palminteri S, Boraud T, Lafargue G, Dubois B, Pessiglione M. Brain hemispheres selectively track the expected value of contralateral options. Journal of Neuroscience. 2009;29:13465–13472. doi: 10.1523/JNEUROSCI.1500-09.2009. [ DOI ] [ PMC free article ] [ PubMed ] [ Google Scholar ]
- Pelli DG. The VideoToolbox software for visual psychophysics: transforming numbers into movies. Spatial Vision. 1997;10:437–442. doi: 10.1163/156856897X00366. [ DOI ] [ PubMed ] [ Google Scholar ]
- Penny WD. Comparing dynamic causal models using AIC, BIC and free energy. NeuroImage. 2012;59:319–330. doi: 10.1016/j.neuroimage.2011.07.039. [ DOI ] [ PMC free article ] [ PubMed ] [ Google Scholar ]
- Penny WD, Stephan KE, Daunizeau J, Rosa MJ, Friston KJ, Schofield TM, Leff AP. Comparing families of dynamic causal models. PLoS Computational Biology. 2010;6:e1000709. doi: 10.1371/journal.pcbi.1000709. [ DOI ] [ PMC free article ] [ PubMed ] [ Google Scholar ]
- Plassmann H, O'Doherty J, Rangel A. Orbitofrontal cortex encodes willingness to pay in everyday economic transactions. Journal of Neuroscience. 2007;27:9984–9988. doi: 10.1523/JNEUROSCI.2131-07.2007. [ DOI ] [ PMC free article ] [ PubMed ] [ Google Scholar ]
- Rangel A, Hare T. Neural computations associated with goal-directed choice. Current Opinion in Neurobiology. 2010;20:262–270. doi: 10.1016/j.conb.2010.03.001. [ DOI ] [ PubMed ] [ Google Scholar ]
- Ratcliff R. A theory of memory retrieval. Psychological Review. 1978;85:59–108. doi: 10.1037/0033-295X.85.2.59. [ DOI ] [ Google Scholar ]
- Ratcliff R, McKoon G. The diffusion decision model: theory and data for two-choice decision tasks. Neural Computation. 2008;20:873–922. doi: 10.1162/neco.2008.12-06-420. [ DOI ] [ PMC free article ] [ PubMed ] [ Google Scholar ]
- Rigoux L, Stephan KE, Friston KJ, Daunizeau J. Bayesian model selection for group studies - revisited. NeuroImage. 2014;84:971–985. doi: 10.1016/j.neuroimage.2013.08.065. [ DOI ] [ PubMed ] [ Google Scholar ]
- Samuelson PA. The Numerical Representation of Ordered Classifications and the Concept of Utility. The Review of Economic Studies. 1938;6:65–70. doi: 10.2307/2967540. [ DOI ] [ Google Scholar ]
- Scheibe C, Ullsperger M, Sommer W, Heekeren HR. Effects of parametrical and trial-to-trial variation in prior probability processing revealed by simultaneous electroencephalogram/functional magnetic resonance imaging. Journal of Neuroscience. 2010;30:16709–16717. doi: 10.1523/JNEUROSCI.3949-09.2010. [ DOI ] [ PMC free article ] [ PubMed ] [ Google Scholar ]
- Shenhav A, Botvinick MM, Cohen JD. The expected value of control: an integrative theory of anterior cingulate cortex function. Neuron. 2013;79:217–240. doi: 10.1016/j.neuron.2013.07.007. [ DOI ] [ PMC free article ] [ PubMed ] [ Google Scholar ]
- Shenhav A, Straccia MA, Cohen JD, Botvinick MM. Anterior cingulate engagement in a foraging context reflects choice difficulty, not foraging value. Nature Neuroscience. 2014;17:1249–1254. doi: 10.1038/nn.3771. [ DOI ] [ PMC free article ] [ PubMed ] [ Google Scholar ]
- Skvortsova V, Palminteri S, Pessiglione M. Learning to minimize efforts versus maximizing rewards: computational principles and neural correlates. Journal of Neuroscience. 2014;34:15621–15630. doi: 10.1523/JNEUROSCI.1350-14.2014. [ DOI ] [ PMC free article ] [ PubMed ] [ Google Scholar ]
- Stephan KE, Penny WD, Daunizeau J, Moran RJ, Friston KJ. Bayesian model selection for group studies. NeuroImage. 2009;46:1004–1017. doi: 10.1016/j.neuroimage.2009.03.025. [ DOI ] [ PMC free article ] [ PubMed ] [ Google Scholar ]
- Stephens DW, Krebs JR. Foraging Theory. Princeton University Press; 1986. [ Google Scholar ]
- Summerfield C, Koechlin E. Economic value biases uncertain perceptual choices in the parietal and prefrontal cortices. Frontiers in Human Neuroscience. 2010;4:208. doi: 10.3389/fnhum.2010.00208. [ DOI ] [ PMC free article ] [ PubMed ] [ Google Scholar ]
- Von Neumann J, Morgenstern O. Theory of Games and Economic Behavior. 1947. [ Google Scholar ]
- Voss A, Nagler M, Lerche V. Diffusion models in experimental psychology: a practical introduction. Experimental Psychology. 2013;60:385–402. doi: 10.1027/1618-3169/a000218. [ DOI ] [ PubMed ] [ Google Scholar ]
- Wagenmakers EJ, van der Maas HL, Grasman RP. An EZ-diffusion model for response time and accuracy. Psychonomic Bulletin & Review. 2007;14:3–22. doi: 10.3758/BF03194023. [ DOI ] [ PubMed ] [ Google Scholar ]
- Wunderlich K, Rangel A, O'Doherty JP. Economic choices can be made using only stimulus values. PNAS. 2010;107:15005–15010. doi: 10.1073/pnas.1002258107. [ DOI ] [ PMC free article ] [ PubMed ] [ Google Scholar ]
- Wunderlich K, Rangel A, O'Doherty JP. Neural computations underlying action-based decision making in the human brain. PNAS. 2009;106:17199–17204. doi: 10.1073/pnas.0901077106. [ DOI ] [ PMC free article ] [ PubMed ] [ Google Scholar ]
- Young CB, Nusslock R. Positive mood enhances reward-related neural activity. Social Cognitive and Affective Neuroscience. 2016;11:934–944. doi: 10.1093/scan/nsw012. [ DOI ] [ PMC free article ] [ PubMed ] [ Google Scholar ]
Decision letter
Collection date 2016.
In the interests of transparency, eLife includes the editorial decision letter and accompanying author responses. A lightly edited version of the letter sent to the authors after peer review is shown, indicating the most substantive concerns; minor comments are not usually included.
Thank you for submitting your article "Frames and biases in the human brain: the impact of prior preferences on decision making" for consideration by eLife . Your article has been reviewed by two peer reviewers, and the evaluation has been overseen by Reviewing Editor Michael Frank and Timothy Behrens as the Senior Editor. The following individuals involved in review of your submission have agreed to reveal their identity: Laurence Hunt (Reviewer #1); Sebastian Gluth (Reviewer #2).
The reviewers have discussed the reviews with one another and the Reviewing Editor has drafted this decision to help you prepare a revised submission.
This manuscript examines the effects of framing choices in terms of a preferred ('default') category of items vs. a less preferred ('alternative') category of items upon choice behaviour (characterised using a drift diffusion model), eye gaze and BOLD fMRI data (with a particular focus on responses in ventromedial prefrontal cortex).
They first show that subjects show a bias towards selecting items from the default category (over and above their preferences expressed before the experiment), both in terms of choices and reaction times. They explain this as a change in starting point of the drift diffusion model. They then use eye-tracking to show that this bias is not explained directly as a consequence of the subjects attending more towards the default item, but instead as a combination of attentional effects on drift rate and a shift in the starting point. Perhaps most strikingly, they show that the default value is present in vmPFC baseline activity, prior to the trial beginning. The key idea is to test whether activity which is often found to reflect chosen minus unchosen value is better thought of as reflecting the value of the default option. They find evidence that this is the case in VMPFC, but not dACC. Overall, this paper addresses a very timely research question, the representation of (choice-related) valuation signals in vmPFC.
Essential revisions:
Both reviewers and the Reviewing Editor were enthusiastic about the contribution, noting that it is a timely approach using a wide array of approaches to address a controversial question, but also noted some essential revisions. These points are amalgamated below.
1) Where does the default bias come from? One possibility is that rather than coming from the decision framing, it comes from a history of choices in favour of that category (of which there will presumably be more towards the default). For instance, if the authors included (chose default(t-1), chose default(t-2), chose default(t-3) etc.) in the logistic regression model, do they see any significant prediction of subjects' choices of the default option on trial t, which decays over trials? Does this reduce the default bias in the constant term?
2) This is also a concern when considering the baseline effect of DV(CAT) in Figure 4A . Is the data at this timepoint being influenced at all by the value of the chosen item, or the value of the default item, on the preceding trial?
3) In Results, the authors conclude from their analyses that "fixation duration […] was not influenced by prior preferences". However, I am not fully convinced by their analysis. They report that people look longer at the default option if it is chosen (with a difference of 81 ms), but they look longer at the other option if this is chosen (with a difference of only 41ms). I think the critical question is whether this 81 vs. 41 ms difference is significant or not. In other words, one could set up an ANOVA with factors "Default vs. Non-Default" and "Chosen vs. Unchosen". Apparently, the "Chosen vs. Unchosen" main effect in such an ANOVA would be significant. Now the question is whether the "Default vs. Non-Default" main effect would also be significant or not. Regarding the same topic: It is mentioned that the graphs in Figure 3C are not significantly different from each other. I was wondering what statistical test the authors used here.
4) Related to point #2, the authors are trying to rule out the Krajbich/Rangel aDDM ideas applying here, in that the default biases cannot be explained just by greater value accumulation for the attended option. My concern is that a simpler model could potentially explain much of the gaze data, whereby there could be a direct effect of gaze on choice – where more looking is predictive of higher probability of choice (regardless of direction of causality) – here, gaze time is additive to the value effects on drift rate, rather than multiplicative as in aDDM. (See Cavanagh et al., 2014 JEP-G for an example of where this additive model provided a better fit than the aDDM; Krajbich/Rangel never tested this simpler model). My worry here is that if subjects attend more to the default option than the non-default option, that this could directly drive drift toward the default. That wouldn't show up as well when fitting the aDDM because it assumes that attention modulates the value, but behavioral data show that there is a bias in choice independent of value, so the authors still need the added starting point bias independent of gaze. But if the subjects just look at the default more, and looking directly predicts choice independent of value, then they might find that the default bias is entirely accounted for by more looking… It would be better if the authors added this direct effect as another model in their model comparison (and perhaps a model with this direct effect plus starting point bias). Still, even if this is the case, I'm not sure it diminishes the contribution in terms of what it tells us about vmPFC or default biases (especially since it would be the default preference that would drive the bias in looking, not the other way around).
5) The authors are keen to show that the RT biases arise directly as a consequence of the choice bias. They provide some evidence of this in Figure 2C . It would be nice to explore this further. One further way of addressing this would be to ask whether the RT bias goes to 0 at the true point of subjective equivalence between the two options (i.e. where P(default) = 0.5, rather than where |VIT(def) – VIT(alt)| =0). Another way of showing it would be to plot Figure 2B without absoluting the X-axis (i.e. plot positive and negative values for VIT(def)-VIT(alt), as in Figure 2A ).
6) A concern when examining the effects in Figure 3C is that any propensity to initially saccade towards the default option might be masked. This could produce a 'starting point' like effect in the aDDM, if one assumes attentional deployment is not random (unlike in the aDDM original formulation). An easy way to show that there was no bias in initial saccade direction would be to show the same plot time-locked to stimulus onset, rather than response.
7) Unsurprisingly, the authors cite Boorman et al., J Neurosci 2013 quite extensively, as this also addressed the question of default vs. alternative coding (but didn't have the nice and extensive behavioural modelling of the present paper). However, the conclusion from Figure 4 seems quite different from that paper. In this paper, dorsal anterior cingulate cortex cares about the chosen vs. unchosen value frame ( Figure 4—figure supplement 1B bottom), whilst VMPFC cares about the default vs. alternative frame ( Figure 4B ). This seemed to me like the opposite conclusion from Boorman's paper (cf. his Figure 4 )…? It would be good for the authors to mention/discuss this.
8) Both reviewers agreed that there was no any information or value that is added to this paper by the decoding analysis. When we already know (from the "standard" analyses) that the vmPFC encodes the value of the default option, it is trivial (almost circular) that we can then also decode preferences from it (it's basically just a question of data quality). Unless the authors can come up with a very convincing argument why this analysis is necessary, they should take it out. Simply doing such an analysis because "we can", and because it is a "fancy" method, is not enough motivation.
Author response
We thank the reviewers for raising this issue, which is indeed important for the interpretation of the default bias. What we showed in the manuscript is that on top of option values, the constant term was significantly positive in the logistic regression used to fit the probability of choosing the default. This constant might capture a prior in favor of the default option, which can be approximated by DV CAT (difference in value between categories), or by the past choices which, on average, should favor the default option. To disambiguate this alternative, we included both DV CAT and past choices (coded 1 for default and -1 for non-default chosen) in the logistic regression. The model was therefore:
logit(Pdef) = Cte + V IT (def) + V IT (alt) + DV CAT + Choice(t-1) + Choice (t-2) etc.
We first noted that when including DV CAT , the constant term was considerably reduced and only bordering significance (from β=0.68±0.13, t(23)=5.40, p=2.10 -5 to β=0.31 ± 0.16, t(23)=1.94, p=0.06). The DV CAT regressor itself was highly significant (β=0.021±0.006, t(23)=3.53, p=2.10 -3 ), suggesting that it was the main factor explaining the default bias. On
the contrary, none of the past choices had a significant effect (all p>0.09, with trends in the opposite direction). In Author response image 1 are illustrated regression estimates obtained with the logistic model that only included the last trial (t-1). Similar results were obtained when including more past trials in the GLM. It therefore appeared that the default bias was not generated by the history of choices.
Author response image 1.
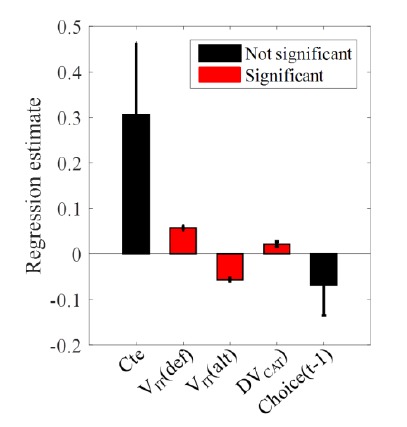
DOI: http://dx.doi.org/10.7554/eLife.20317.009
This analysis is now mentioned in the Results section of the manuscript, as follows:
“Crucially, the constant was significantly positive (β=0.68±0.13, t(23)=5.40, p=2.10 -5 ), bringing evidence for a bias toward the default option. […] Consistently, the constant estimate was not different when restricting the logistic regression to the first choice in a block (β first =0.58 ± 0.49, β all =0.68±0.13, difference: t(23)=0.97 p=0.44), confirming that the default bias was not resulting from the history of past choices.”
We understand the reviewer’s concern: the problem is that DV CAT might be correlated with the default or chosen option value of the preceding trial. We ran another version of GLM2 with the parametric modulators (V IT (def_chosen), V IT (alt_unchosen), V IT (def_unchosen), V IT (alt_chosen)) replaced by the same values but for the options presented in the previous trial (the first trial of each block was discarded). In order to correct for DV CAT , we also included DV CAT as a parametric modulator before the four item values. We did not find any significant effect of previous trial option values in baseline activity (all p>0.1 for the 4 values at the 2 time points t=-2 and t=0 sec from stimulus onset). Thus, it seems that the values of the options presented in the preceding trial (default or alternative, chosen or not) do not significantly influence vmPFC baseline activity, beyond the shared variance with DV CAT .
We have also mentioned this analysis in the Results section of the manuscript, as follows:
“As we realized that the evoked response might have contaminated baseline vmPFC activity at the next trial, we estimated another version of GLM2, with the four parametric modulators – V IT (def_chosen), V IT (alt_unchosen), V IT (def_unchosen), V IT (alt_chosen) – replaced by the same values but for the options presented in the previous trial (the first trial of each block was discarded). None of these parametric modulators had a significant effect at the time points preceding option display (t=-2 and t=0 s from stimulus onset). Thus, the values of the options presented in the previous trial did not affect baseline vmPFC activity, beyond the variance that they shared with DV CAT .”
We have run the ANOVA suggested by the reviewer, which confirmed our interpretation: there was a significant effect of the ‘Chosen vs. Unchosen’ factor, but no significant effect of the ‘Default vs. Alternative’ factor. We note that the reviewer is concerned with the interaction, which was indeed significant (see below).
The reasons why we did not comment on the interaction is because 1) the two factors are not orthogonal (the default option is more frequently chosen) and 2) it is confounded by the effect of option values on response time (hence total fixation time).
In order to further investigate this interaction and to take into account the effect of DV IT , we plotted the proportion of fixation for the default option as a function of DV IT , separately for the two types of choices (Black: default chosen; Red: default unchosen). Author response image 2 shows that when controlling for decision value, the two situations are symmetrical: the advantage of the chosen option is similar whether that chosen option is the default or the alternative. Thus, the interaction might result from the fact that decision value is on average larger when the default is chosen, yielding a bigger advantage for fixation of the chosen option in that case. Note that the proportions of fixations are symmetrical around the point of subjective equivalence (E) between the two options computed from choices.
Author response image 2.
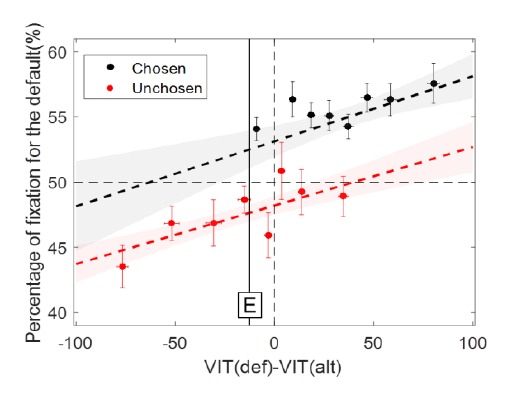
DOI: http://dx.doi.org/10.7554/eLife.20317.010
We have not added this figure in the revised manuscript as we think this interaction on gaze fixations is rather anecdotal relative to the main conclusions of our study. However, we have incorporated the ANOVA in the Results section, as follows:
‘To test the link between prior preference and gaze fixation pattern, we compared the duration of fixation for the default and alternative options, separately for trials in which the default was chosen and unchosen. […] The interaction was significant (F(1,88)=17.45, p=1.10 -4 ), reflecting the fact that the default option was more frequently chosen, and with larger decision values.”
We have fitted the model suggested by the reviewers, with an additive and not multiplicative effect of gaze on the drift rate. As can be seen in Author response image 3 , the additive model could not account for the default bias (left panel), such that we still needed the shift in the starting point (right panel).
Author response image 3.
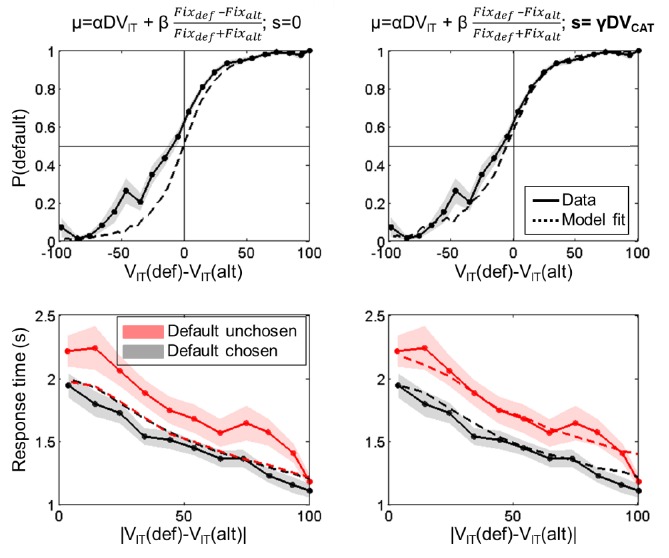
DOI: http://dx.doi.org/10.7554/eLife.20317.011
In fact, the additive model was doomed to fail since it was not the case that participants look at the default more. We have nonetheless mentioned this additional analysis in the Results section:
“Although using the fixation pattern (with θ) improved the fit, only the prior preference (with S) could explain the decision bias toward the default option. We reached similar conclusions when the advantage for the attended option was additively included in the drift rate, on top of decision value (as in Cavanagh et al., 2014). In fact, gaze fixation pattern failed to produce the default bias simply because the default option was no more looked at than the alternative option.”
We think there is a confusion here. We do not claim that the RT bias arises directly as a consequence of the choice bias. We claim that choice and RT biases have the same origin – the prior preference between categories, which is quantified by DV CAT . The prediction that follows on this claim is that choice and RT biases should both go to 0 when DV CAT goes to 0. This is verified in the correlation across blocks (note that DV CAT varies across blocks) shown in Figure 2C . It is also verified in the model comparison showing that taking DV CAT as a starting point in the DDM produces both the choice and RT biases.
This does not imply that the RT bias should go to 0 at the point of subjective equivalence, i.e. when the decision value DV IT (not DV CAT ) is null. We have checked that the DDM does not make this prediction, using simulations ( Author response image 4 ). In simulation (1) we fixed the starting point at 0 and we vary the drift rate from -0.1 to 0.1. In this case, corresponding to DV CAT =0, there was no bias in choices or in RT. In simulation (2) the starting point was fixed to 0.1 and the drift rate varied as in (1). In this case, we did see a bias in both choice and RT. From simulation (2), we computed the ‘true point of subjective equivalence’ (E=-0.013) for which Pdef=0.5. Then we ran simulation (3), with a starting point still fixed at 0.1 and the drift varying from -0.1-E to 0.1-E. We found that the choice bias was cancelled but that the RT bias survived, including at the equivalence point (when drift was equal to –E).
Author response image 4.
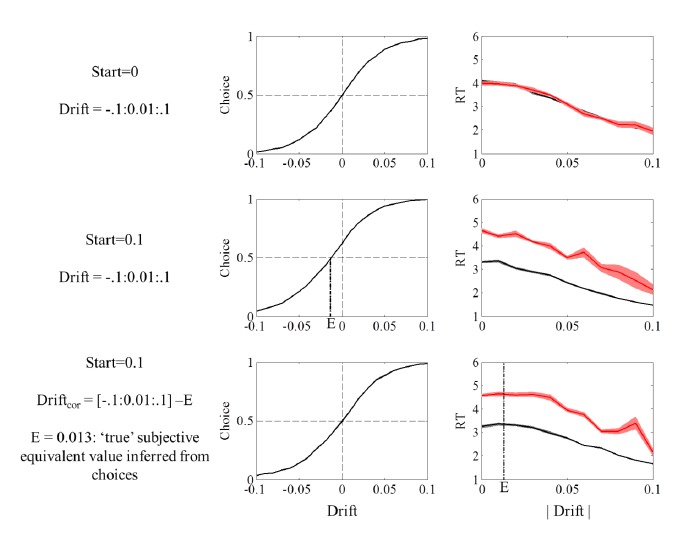
DOI: http://dx.doi.org/10.7554/eLife.20317.012
Thus, it should not be expected that taking equivalent subjective values at the item level would cancel the RT bias induced by the preference at the category level. Consistently, we observed in our data that when item values were corrected so as to eliminate the choice bias, the RT bias was still significant (t(23)=8.43, p=4.10 -4 ).
We have not included these analyses in the revised manuscript as there was no reason to predict a cancellation or the RT bias with DV IT =0, and because it seems rather distractive from the main claim which is about the effect of prior preference (DV CAT ).
We nonetheless inserted a comment on the fact that choice and RT biases tended to vanish when DV CAT approached zero (Results section:
“We verified this conclusion by testing the correlation across blocks between the posterior means of the 12-free-S model and the prior preference DV CAT ( Figure 2F ). The correlation was significant at the group level (r=0.35 ± 0.07, t(23)=5.12, p=3.10 -5 ), strengthening the idea that prior preference was imposing a shift in the starting point that resulted in both choice and RT biases. Thus, the correlation observed between choice and RT biases was driven by variations in DV CAT across blocks, the two biases tending to vanish when DV CAT approached zero.”
When investigating the time course of gaze fixations locked to stimulus onset, we found a strong bias in the initial saccade toward the left option but not toward the default option (see left graph in Author response image 5 , in which stars indicate time points surviving correction for multiple comparisons obtained through permutation tests). Actually, the initial fixation pattern was essentially spatial: subjects looked first left and then right. The preferential looking of the default option emerged later, corresponding to the fact that it was more frequently chosen. This result is confirmed in the right panel showing that the proportion of fixations between 0 and 200 ms is significantly different from 50% for the left option (t(22)=13.3, p=6.10 -12 ) but not for the default option (t(22)=1.28, p=0.21).
Author response image 5.
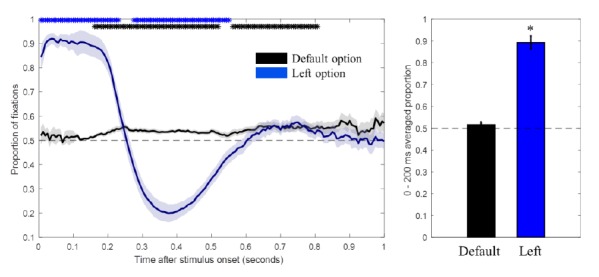
DOI: http://dx.doi.org/10.7554/eLife.20317.013
We have included this observation in the revised manuscript (Results section), as follows:
“Thus, fixation duration was indeed predictive of choice, but was not influenced by prior preference. […] This result was further confirmed by a model comparison showing that fixation duration for each option was better explained (xp=0.999) by a GLM including the unsigned decision value and the choice (chosen vs. unchosen option) than by GLMs including an additional regressor that indicated the prior preference (default vs. alternative option).”
7) Unsurprisingly, the authors cite Boorman et al., J Neurosci 2013 quite extensively, as this also addressed the question of default vs. alternative coding (but didn't have the nice and extensive behavioural modelling of the present paper). However, the conclusion from Figure 4 seems quite different from that paper. In this paper, dorsal anterior cingulate cortex cares about the chosen vs. unchosen value frame ( Figure 4—figure supplement 1B bottom), whilst VMPFC cares about the default vs. alternative frame ( Figure 4B ). This seemed to me like the opposite conclusion from Boorman's paper (cf. his Figure 4 )? It would be good for the authors to mention/discuss this.
The reviewer is absolutely correct: our results stand in contradiction to those reported in Boorman et al., J Neurosci 2013. The conclusions of this paper are indeed that decision values are signaled in the choice frame by the vmPFC, and in the default frame by the dACC. The reason why we did not discuss the contradiction is that we had no good explanation to provide. When taking a closer look at that paper, we realized that the result regarding dACC was not so clear-cut (see their Figure 4 ). The result regarding vmPFC clearly suggested a post- choice framing, but the activation peak arose much later than what we observed (10 sec vs. 4- 6 sec after option display). It could be that subjects in their design did not set up a default as we define it, which implies anticipating a default response, associated with shortening of response times (RT are not reported in their paper). This would explain why value coding appeared long after the choice was made, and why it was expressed in a post-choice frame only. We now mention the discrepancy and this difference of timing in the Discussion:
“The analysis of the evoked response showed that the vmPFC and ventral striatum encode the decision value in a frame that opposes the default to the alternative option. […] Accordingly, the representation of chosen option value was largely delayed in comparison to our results (peaking 10s after option display), possibly related to the necessity of storing expected values in a learning context.”
The motivation was not that ‘we can’ or ‘it is ‘fancy’, but simply pedagogic. In our experience when presenting this study, Figure 5 helps a lot to understand the link between prior preference and comparison frame. We nonetheless agree that it provides no additional evidence, so we removed the parts regarding decoding analysis from the Methods, Results, Discussion and figure/legend sections.
Associated Data
This section collects any data citations, data availability statements, or supplementary materials included in this article.
Supplementary Materials
- View on publisher site
- PDF (2.2 MB)
- Collections
Similar articles
Cited by other articles, links to ncbi databases.
- Download .nbib .nbib
- Format: AMA APA MLA NLM
Add to Collections

IMAGES
COMMENTS
Jun 4, 2007 · Prior Hypothesis bias refers to the fact that decision makers who have strong prior beliefs about the relationship between two variables tend to make decisions on the basis of those beliefs, even when presented with the evidence that their beliefs are wrong.
Prior hypothesis bias cognitive bias that is a result from the tendency to base decisions on strong prior beliefs even if evidence shows that those beliefs are wrong. 1 / 37
May 14, 2016 · Prior hypothesis 2. Availability 3. Confirmation. 4. ... It’s this preferential mode of behaviour that leads to the confirmation bias. ... Another example, ...
Oct 8, 2024 · Study with Quizlet and memorise flashcards containing terms like 1. A cognitive bias resulting from the tendency to base decisions on strong prior beliefs even if evidence shows that those beliefs are wrong is known as:, 2. A cognitive bias resulting from the tendency to generalize inappropriately from a small sample or from a single vivid event or episode is known as:, 5. A decision maker's ...
cognitive bias resulting from the tendency to base decisions on strong prior beliefs even if the evidence shows that those beliefs are wrong. Representativeness bias Cognitive bias resulting from tendency to generalize inappropriately from a small sample or from a single vivid event or episode
Jun 22, 2023 · Psychological theories vary in defining memory bias. Some theories state that information confirming prior beliefs is stored in the memory while contradictory evidence is not (i.e., Schema theory). Some others claim that striking information is remembered best (i.e., humor effect). Memory confirmation bias also serves a role in stereotype ...
Therefore, our hypothesis implies that prior preferences should (1) induce a bias in favor of the default option, and (2) determine the frame of the value comparison process. The purpose of the present study was to examine how these two constraints would shape the brain valuation signal.
It is an issue that is commonly overlooked in health settings for a variety of reasons, such as having no real-world data to detect or correct for hypothetical bias.[4] Example. Buckell and Hess (2019) use an online DCE in the US tobacco market, and US tobacco market data, to show the presence of (and correct for) hypothetical bias.[5]
Prior Hypothesis Bias = Occurs when decision-makers make decisions based on strong prior beliefs despite evidence Escalating Commitment = Occurs when decision makers commit more resources to a failing project after already investing resources Representativeness = Rooted in the tendency to generalize from a small sample or vivid anecdote Illusion of Control = Rooted in the tendency to ...
Jun 17, 2019 · This is the same as adding a prior for the model parameter (for example, ridge regression is equivalent to linear regression with a normal prior, of mean zero and certain variance, for parameters). A prior is used to incorporate null hypothesis/expert opinion/theoretical insights on the distribution of model parameters.